
Overdrainage means that too much CSF is being discharged, leading to low intraventricular pressure and sometimes even suction in the ventricles.
The outwardly-visible acute symptoms are largely similar to those associated with underdrainage and include headaches, vertigo and nausea. At first, it is difficult to tell the difference between the two types of complication.
However, in cases of overdrainage, unlike with underdrainage, the imaging may show that the ventricle has shrunk or collapsed completely – a condition known as “slit ventricle syndrome”.
Slit ventricles do not have any pathological significance per se. The term simply refers to the width of the cerebral ventricles that narrow into slits due to overdrainage. However, if, for example, the CT or MRI scan appears to show this issue it will confirm a suspected diagnosis of "overdrainage".
INTRAVENTRICULAR PRESSURE
Intraventricular Pressure (IVP) is the differential pressure relative to the external atmospheric pressure. “Negative IVP” or suction therefore occurs when the pressure in the ventricles is lower than the atmospheric pressure.
Overdrainage can put brain tissue under significant pressure. However, this is not caused by compression, but by tensile stress, which pulls the surface of the cerebral cortex away from the cranial wall, towards the ventricle. There have even been documented cases in which this suction has caused the brain stem or cerebellum to move, bending the very narrow aqueduct (an important channel that connects the third and fourth ventricle).
Nevertheless, these imaging techniques often do not immediately lead to such a clear diagnosis, if they even lead to any diagnosis at all. In fact, they often appear unremarkable: the aforementioned morphological changes to the ventricle and the brain take time to develop. Naturally, this also depends on the severity of the overdrainage and the compliance of the brain.
Compliance
“Compliance” is an important term in the field of hydrocephalus therapy. This is not only typical in the brain, but also affects the entire central nervous system (CNS), i.e. the cranial and spinal cavities. Almost all of the space in these areas is taken up by brain tissue and the spinal cord (approximately 1,400 ml). They also contain arterial and venal blood (approximately 150 ml in total, at a ratio of 1:2), as well as CSF (approximately 150 ml).
To give a clearer description, compliance reflects something like the “softness” or “elasticity” of the entire contents of the cranial and spinal cavities. This elasticity is a type of “pseudo-elasticity”, due to the fact that the elements contained in the cranial/spinal cavity, such as blood and CSF, are inherently incompressible, or, in other words, rigid. Compliance comes about due to the fact that with every new “mass”, e.g. a buildup of excess CSF (hydrocephalus), a bleed (haematoma) or a growth (cancer, cysts or swelling), venal blood is essentially displaced from the rigid space and forced out into the body. Therefore, if compliance is normal, such masses will only cause a slight increase in pressure within the skull (intracranial pressure or ICP) to begin with, which is why they are often associated with a so-called "pressure reserve capacity”. However, when the mass becomes too large the reserve capacity quickly gets used up and the ICP increases sharply. However, masses that are too large are not the only reason for reduced compliance. In fact, this can also be caused by a pathological hardening of the tissue and blood vessels, particularly the veins (sclerosis). Measuring compliance can also be said to have “diagnostic value”, as it makes it possible to find out some information regarding the size of the mass, as well as the state of the tissue and blood vessels.
The precise medical definition of compliance in the form of a pressure to volume ratio (C = dV/dP) is set out in the following pressure-volume curve:
A certain physiological (natural/healthy) mass in the cranial cavity is caused by the wave-like inflow of arterial blood. The following diagram shows how the amplitude (i.e. the level) of the constant ICP pulse waves depends on the level of compliance: a high ICP wave amplitude shows a reduced level of compliance.
Although only a small percentage of the total CSF is contained in the bony spinal canal, this means it contains a greater amount of venal blood. The dural sac, which contains the spinal CSF, is flexible and expands significantly when the person stands, due to the CSF sinking down from the skull. The spinal canal probably accounts for more than 50% of total compliance.
Overdrainage is not as easy to explain as underdrainage. How can such a large amount of suction be produced just by implanting a shunt, i.e. artificially linking the ventricle with the abdomen (as is usually the case)? The only place in the human body in which there is a certain level of moderate negative pressure (suction) is the pleural gap (p = approx. - 5cmH2O), but this is rarely considered for use as an outflow for drainage anymore. In the past, there was also speculation about a “dynamic pump mechanism” e.g. in crying children. However, this could not be confirmed.
However so-called hydrostatic pressure (HSP) could provide a satisfactory and plausible explanation. This only occurs when the patient is in a standing position and is caused by the weight of the column of liquid in the shunt (i.e. particularly in the long peritoneal catheter). At the bottom end of the catheter this weight becomes noticeable due to the weight pressure placed on the column of liquid. However, there is suction at the upper end of the catheter in the form of hydrostatic pressure (HSP) or hydrostatic suction, depending on the reference point. Without a valve, the column of liquid would simply flow through the open catheters at the top and bottom, with the process being driven by the weight of the liquid. In other words: if the patient stands up straight and the ventricles above are connected to the abdomen below via the long catheter, they will "want" to empty all the fluid out. Another way to describe this to say the ventricles are "sucked dry", which is why the term "siphoning" is often used to describe this effect. However, this extreme scenario would only occur if external air were able to flow through the ventricle. However, given that the ventricles are usually closed, the CSF only keeps draining away until the hydrostatic suction is balanced out by the tensile stress that occurs in the brain tissue ("counter suction"). The degree to which this will occur in a specific situation depends, inter alia, on the elasticity of the tissue (compliance) and the amount of suction.
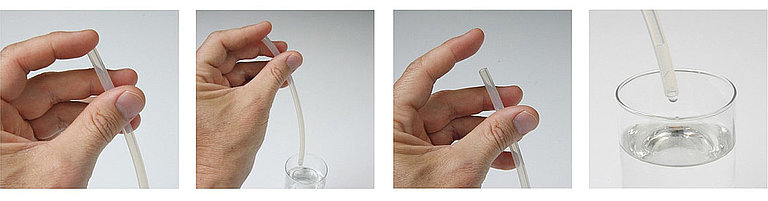
Hydrostatic pressure in cmH2O
To use technical jargon, pressure in the CFS is measured using the non-SI unit "cmH2O" ("SI" stands for Système international d'unités or the "International System of Units"). This unit is otherwise known as the "centimetre of water". This is a type of so-called "differential pressure", which is used to describe the difference in pressure between the cranial cavity and the external air pressure.
cmH2O is defined as the hydrostatic pressure exerted by a one-centimetre-high column of water, in which the density of the water is precisely one gram per cubic centimetre. It is expressed differently to the unit "Pa", which is normally used to measure pressure. Unlike a "Pascal", which is defined as the force of one Newton applied to an area of one cubic metre, the cmH2O is a unit that does not have to refer to an effective surface area. It only refers to a one-dimensional measure of length, namely the height of the column of water exerting force on the relevant pressure point. This goes against our intuitive understanding of pressure, however, in doing so, it makes the effect of hydrostatic pressure (i.e. the pressure in a medium, such as a liquid, that is generated by the weight of the medium itself) clearer. In other words: the pressure is generated because the medium is pressing against itself with its own weight.
One cmH2O corresponds roughly to one millibar, or, in SI units: 1,000 Pascal.
Hydrostatic pressure can be calculated using the following formula:
HSP = rho * g * h
, in which h (the height of the column of liquid), rho and g are physical constants.
Therefore, in the absence of other factors, the suction in an average adult can reach 50 cmH2O. The tapering of the ventricle produces this high amount of tensile stress due to the fact that the exterior surface of the brain is firmly attached to the skull and so, as a whole, cannot "shrink".
According to this physical formula, the effect will be reduced when a person is lying down, due to the fact that the height of the column will naturally be h = 0 cm, in turning meaning that HSP = 0. That is clearly very plausible, due to the fact that the column of liquid in a catheter that is "lying down" (horizontal) will no longer be squeezed out the end under the force of its own weight. In other words: the ventricle connected to the abdomen will no longer be at risk of being sucked dry either. In such a situation, there will no longer be any "siphoning effect".
It must again be emphasised that this physical effect will ALWAYS occur and is UNAVOIDABLE when the patient stands. When this happens, a huge suction force of 20 - 60 cmH2O will be produced, with the severity depending on the height of the patient or the distance between the ventricle and the diaphragm. The symptoms described above, such as slit ventricle syndrome and the movement of entire areas of the brain can be caused by this alone. Even this purely physical explanation makes it clear, that unlike underdrainage, the very similar symptoms caused by overdrainage ONLY occur when the patient is standing up, and will subside again relatively quickly once the patient lies down. This provides a good diagnostic criterion for when attempting to distinguish one of these phenomena from the other.
Still, low intraventricular pressure is not the primary issue when it comes to overdrainage. Rather, the primary issue is the fact that the expansion of the brain tissue can lead to the formation of hygromas and haematomata (i.e. cavities filled with liquid and blood) particularly when this occurs between the surface of the brain and the skull. The brain is firmly attached to the skull via the intricately-structured arachnoid mater and dura mater, which it usually cannot be detached from. If it is sucked, away from the cranial wall, with too much force these delicate membranes and the small bridging veins running through them will be the first things to rip and start bleeding. However, large venous sinuses in the dura or arteries can also be damaged. Overdrainage almost exclusively causes subdural haematomata (SDH). There is even the possibility that these may become chronic. Laboratory experiments have shown how a typical level of hydrostatic suction in the ventricles of around 30 cmH2O can subject the external membranes of the brain to forces equivalent to several hundred grams in weight. The scenario described above makes it clear how haematomata caused by overdrainage can become chronic (chronic subdural haematomata or cSDH).
Another equally serious problem induced by overdrainage is the so-called slit ventricle syndrome (SVS). Suction causes the ventricles to narrow, which can close the fine openings of the ventricular catheter (VC). The cerebrospinal fluid can then no longer be drained and the ventricles dilate again. If the suction then takes effect again, the process starts all over again. This periodic contraction and expansion of the ventricles, which is associated with strong tension, can already damage the tissue. If tissue then grows into the small catheter holes, this can lead to further tissue tears and bleeding during the subsequent ventricular expansion. If the ventricular catheter becomes irreversibly blocked, the ventricles remain narrow and shunt revision becomes unavoidable. As early as 1987, Rolf Gruber described this phase of SVS as follows:
"In shunt revision, the fixed ventricular catheter can only be removed with difficulty; its lumen is completely or partially obstructed by incarcerated tissue plaques in the ostia. They consist of vascularized neuroglia, plexus components and blood clots. Ependymal cells are only found on the torn tissue fragments outside, but never inside the catheter." [Gruber3, Das Schlitz-Ventrikel-Syndrom, 1987].
To this day, many authors assume that these demonstrable injuries to the ependyma due to chronic overdraining (i.e. not only in children) lead to a permanent stiffening/hardening of the ventricular walls and an associated reduction in brain compliance ("stiff ventricles"). This hypothesis is supported by the fact that the ventricles do not necessarily return to their original ventricular width once physiological, i.e. "normal" pressure conditions have been restored [Pollay4 1994, Ros5 2018, Wagner6 2018]. However, investigations into the contribution of pathological cell changes to this presumed ventricular stiffening have not yet been able to provide definitive clarity [Oi9 1986, Del Bigio7 2002, Di Rocco8 2015 ].