Over- and underdrainage
- the most common “side effects” of shunt systems
A look back at the last 70 years of over- and underdrainage in shunts
Over- and underdrainage are inextricably linked to the normal physical functioning of shunts. For instance, neither problem is the result of malfunctions caused by negligence or a lack of understanding. Rather, such problems are an inevitable result of using a shunt to create an artificial, unphysiological link between body cavities that are usually separate from one-another. That is why it is so important to be familiar with the basic principles of both phenomena in terms of physics, meaning that they can be treated with the right combination of treatment and technology.
show more"A shunt is like a horse harnessed to a broken-down car."
There are many different ways in which hydrocephalus can manifest itself and it has many different causes. Although people have been aware of the symptoms since ancient times, most of the causes, to use scientific language, are “not particularly well understood”. There are even some that are completely unknown. It is difficult to tackle causes that are unknown or not understood. At the same time, this condition is far from rare and can affect any age group.
Even today, it is only treated symptomatically. This involves reducing the dangerous overpressure in the patient’s skull using a valve and draining it into another suitable body cavity (often in the abdomen).
SHUNT SYSTEMS
A shunt system usually consists of a (proximal) ventricular catheter, a (frontal or occipital) valve to regulate the opening pressure and a (distal) catheter for draining it into a particular body cavity (the right atrium of the heart or the abdomen). A shunt system like this can also be equipped with a reservoir (burr hole reservoir or prechamber).
The first shunt was implanted almost exactly 70 years ago (in 1949) in Philadelphia by the neurosurgeons Spitz and Nulsen. It was so basic and prone to failure that, as far as we know, it only aided a single patient for a short time. Six years later, the valve improved significantly by the locksmith, John Holter, meaning that most people view the “shunt therapy era” as only beginning in 1955. The famous story of how John Holt tried desperately to fight for the life of his newborn son (ultimately in vain, sadly), who was suffering from hydrocephalus is set out in the following, very well-written, account: HOLTER’S BRAIN DRAIN.
Since then, the basic principle of “shunting” has remained the same, which is unusual in the field of medicine. However, from a purely technological standpoint, there have been many significant improvements. Today, high-quality shunts are made from durable, biocompatible materials to ensure that they do not break, corrode or trigger a rejection reaction. Antibacterial catheters can reduce rates of infection to less than 5% (Ritz1, 2007; Parker2, 2015) and opening pressures can be adjusted to suit the specific needs of the patient. Modern shunts like this allow many patients to lead a long life with barely any restrictions, which really does represent a major step forward.
However, despite fantastic modern shunts, the continued lack of knowledge about the causes of hydrocephalus has lead to problems when treating the condition.
To illustrate things better, perhaps it would be useful to compare the shunt with a horse tied to a car that has a faulty engine. Even though the no-one really knows the reason for the engine damage, the car will move again (even if it is a bit slower) and will therefore be able to reach its original destination. It does not matter whether the problem has been caused by a tear in the fuel line, a broken engine mount or a defective spark plug, as the method will almost always work for the time being. You just have to follow a few new rules: e.g. feeding the horse regularly and giving it breaks. AND: Now new, completely different things can suddenly go wrong. The horse could simply gallop off down a rough track, pulling the car in the wrong direction or even drag it into the ditch. The driver, who before only had to know about his car, now has to be able to drive the horse and learn to understand it.
For example, a shunt can get blocked over time, and, even today, implanting a new one is often the only available solution. So-called “siphoning” is another particularly unpleasant, but unavoidable side-effect: the laws of physics mean that when a person is standing, a high level of suction (or negative pressure) is produced in the ventricles, between the head and abdomen, due to the fact that gravity causes the liquid in the catheter to be drawn downwards. This suction poses as great a danger to the brain as the original overpressure (hydrocephalus) can give rise to brain haemorrhages. (The following article provides more detailed information on this phenomenon: "Foundations of fluid mechanics, or of beakers, bottles and people?"
It is not possible to stave off this side effect using a normal shunt: special techniques are required to counteract it.
Underdrainage is therefore inextricably linked to the normal physical functioning of the shunt, as is overdrainage, which is particularly dangerous. For instance, neither problem is the result of malfunctions caused by negligence or a lack of understanding. Rather, such problems are an inevitable result of using a shunt to create an artificial, unphysiological link between body cavities that are usually separate from one-another.
It is almost impossible to prevent either overdrainage or underdrainage when implanting a single differential pressure valve. This procedure is just as common today as was in the past. The only thing the responsible doctor can do is to cleverly configure the valve in a way that constitutes a "clinically acceptable" compromise between these two issues, under the circumstances. Simply implanting another valve to counteract the physical forces responsible for overdrainage, and the occasionally dramatic effects it has on the patient, is something that is important to avoid. This will be explained in the section below.
Underdrainage means that too little CSF is being discharged through the shunt. The pathological overpressure in the ventricles goes back to roughly the way it was before or is not sufficiently reduced. Some or all of the original symptoms of hydrocephalus, including headaches, vertigo and nausea will then return. Children may also experience changes in their facial expression (sunset eyes, papilloedema) and a gradual increase in head circumference. The cardinal symptoms that affect normal pressure hydrocephalus (NPH) patients are incontinence, symptoms of confusion similar to dementia and the typical gait abnormalities, causing the patient to take small steps. These outwardly-visible symptoms will be constant: to be more specific, they will occur regardless of whether the patient is standing or lying down.

Sometimes, the MRI or CT imaging will show that the overpressure caused by underdrainage is leading the ventricles to dilate, compressing the brain tissue and pushing it against the cranial wall from the inside. This destroys nerve cells and causes them to die-off. The process takes place slowly, over the course of weeks and months, depending on the level of overpressure. Nevertheless, in the long term it is irreversible, due to the fact that the nerve cells cannot be regenerated.
Underdrainage can easily be explained by valves in which the flow-resistance is too high or the opening pressure is too high: they are simply not fulfilling their intended therapeutic function. In such a case, if an adjustable valve has been implanted, the first countermeasure should of course be opening it up. This should be done while the amount of overpressure is still low, so that the CFS to be drained early on. If this does not lead to any improvement, the valve may be blocked, meaning that a revision will need to be carried out.

Overdrainage means that too much CSF is being discharged, leading to low intraventricular pressure and sometimes even suction in the ventricles.
The outwardly-visible acute symptoms are largely similar to those associated with underdrainage and include headaches, vertigo and nausea. At first, it is difficult to tell the difference between the two types of complication.
However, in cases of overdrainage, unlike with underdrainage, the imaging may show that the ventricle has shrunk or collapsed completely – a condition known as “slit ventricle syndrome”.
Slit ventricles do not have any pathological significance per se. The term simply refers to the width of the cerebral ventricles that narrow into slits due to overdrainage. However, if, for example, the CT or MRI scan appears to show this issue it will confirm a suspected diagnosis of "overdrainage".
INTRAVENTRICULAR PRESSURE
Intraventricular Pressure (IVP) is the differential pressure relative to the external atmospheric pressure. “Negative IVP” or suction therefore occurs when the pressure in the ventricles is lower than the atmospheric pressure.
Overdrainage can put brain tissue under significant pressure. However, this is not caused by compression, but by tensile stress, which pulls the surface of the cerebral cortex away from the cranial wall, towards the ventricle. There have even been documented cases in which this suction has caused the brain stem or cerebellum to move, bending the very narrow aqueduct (an important channel that connects the third and fourth ventricle).
Nevertheless, these imaging techniques often do not immediately lead to such a clear diagnosis, if they even lead to any diagnosis at all. In fact, they often appear unremarkable: the aforementioned morphological changes to the ventricle and the brain take time to develop. Naturally, this also depends on the severity of the overdrainage and the compliance of the brain.
Compliance
“Compliance” is an important term in the field of hydrocephalus therapy. This is not only typical in the brain, but also affects the entire central nervous system (CNS), i.e. the cranial and spinal cavities. Almost all of the space in these areas is taken up by brain tissue and the spinal cord (approximately 1,400 ml). They also contain arterial and venal blood (approximately 150 ml in total, at a ratio of 1:2), as well as CSF (approximately 150 ml).
To give a clearer description, compliance reflects something like the “softness” or “elasticity” of the entire contents of the cranial and spinal cavities. This elasticity is a type of “pseudo-elasticity”, due to the fact that the elements contained in the cranial/spinal cavity, such as blood and CSF, are inherently incompressible, or, in other words, rigid. Compliance comes about due to the fact that with every new “mass”, e.g. a buildup of excess CSF (hydrocephalus), a bleed (haematoma) or a growth (cancer, cysts or swelling), venal blood is essentially displaced from the rigid space and forced out into the body. Therefore, if compliance is normal, such masses will only cause a slight increase in pressure within the skull (intracranial pressure or ICP) to begin with, which is why they are often associated with a so-called "pressure reserve capacity”. However, when the mass becomes too large the reserve capacity quickly gets used up and the ICP increases sharply. However, masses that are too large are not the only reason for reduced compliance. In fact, this can also be caused by a pathological hardening of the tissue and blood vessels, particularly the veins (sclerosis). Measuring compliance can also be said to have “diagnostic value”, as it makes it possible to find out some information regarding the size of the mass, as well as the state of the tissue and blood vessels.
The precise medical definition of compliance in the form of a pressure to volume ratio (C = dV/dP) is set out in the following pressure-volume curve:
A certain physiological (natural/healthy) mass in the cranial cavity is caused by the wave-like inflow of arterial blood. The following diagram shows how the amplitude (i.e. the level) of the constant ICP pulse waves depends on the level of compliance: a high ICP wave amplitude shows a reduced level of compliance.
Although only a small percentage of the total CSF is contained in the bony spinal canal, this means it contains a greater amount of venal blood. The dural sac, which contains the spinal CSF, is flexible and expands significantly when the person stands, due to the CSF sinking down from the skull. The spinal canal probably accounts for more than 50% of total compliance.
Overdrainage is not as easy to explain as underdrainage. How can such a large amount of suction be produced just by implanting a shunt, i.e. artificially linking the ventricle with the abdomen (as is usually the case)? The only place in the human body in which there is a certain level of moderate negative pressure (suction) is the pleural gap (p = approx. - 5cmH2O), but this is rarely considered for use as an outflow for drainage anymore. In the past, there was also speculation about a “dynamic pump mechanism” e.g. in crying children. However, this could not be confirmed.
However so-called hydrostatic pressure (HSP) could provide a satisfactory and plausible explanation. This only occurs when the patient is in a standing position and is caused by the weight of the column of liquid in the shunt (i.e. particularly in the long peritoneal catheter). At the bottom end of the catheter this weight becomes noticeable due to the weight pressure placed on the column of liquid. However, there is suction at the upper end of the catheter in the form of hydrostatic pressure (HSP) or hydrostatic suction, depending on the reference point. Without a valve, the column of liquid would simply flow through the open catheters at the top and bottom, with the process being driven by the weight of the liquid. In other words: if the patient stands up straight and the ventricles above are connected to the abdomen below via the long catheter, they will "want" to empty all the fluid out. Another way to describe this to say the ventricles are "sucked dry", which is why the term "siphoning" is often used to describe this effect. However, this extreme scenario would only occur if external air were able to flow through the ventricle. However, given that the ventricles are usually closed, the CSF only keeps draining away until the hydrostatic suction is balanced out by the tensile stress that occurs in the brain tissue ("counter suction"). The degree to which this will occur in a specific situation depends, inter alia, on the elasticity of the tissue (compliance) and the amount of suction.
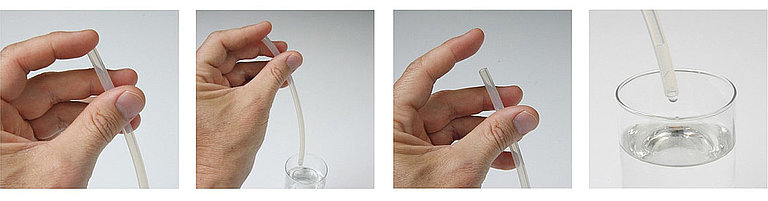
Hydrostatic pressure in cmH2O
To use technical jargon, pressure in the CFS is measured using the non-SI unit "cmH2O" ("SI" stands for Système international d'unités or the "International System of Units"). This unit is otherwise known as the "centimetre of water". This is a type of so-called "differential pressure", which is used to describe the difference in pressure between the cranial cavity and the external air pressure.
cmH2O is defined as the hydrostatic pressure exerted by a one-centimetre-high column of water, in which the density of the water is precisely one gram per cubic centimetre. It is expressed differently to the unit "Pa", which is normally used to measure pressure. Unlike a "Pascal", which is defined as the force of one Newton applied to an area of one cubic metre, the cmH2O is a unit that does not have to refer to an effective surface area. It only refers to a one-dimensional measure of length, namely the height of the column of water exerting force on the relevant pressure point. This goes against our intuitive understanding of pressure, however, in doing so, it makes the effect of hydrostatic pressure (i.e. the pressure in a medium, such as a liquid, that is generated by the weight of the medium itself) clearer. In other words: the pressure is generated because the medium is pressing against itself with its own weight.
One cmH2O corresponds roughly to one millibar, or, in SI units: 1,000 Pascal.
Hydrostatic pressure can be calculated using the following formula:
HSP = rho * g * h
, in which h (the height of the column of liquid), rho and g are physical constants.
Therefore, in the absence of other factors, the suction in an average adult can reach 50 cmH2O. The tapering of the ventricle produces this high amount of tensile stress due to the fact that the exterior surface of the brain is firmly attached to the skull and so, as a whole, cannot "shrink".
According to this physical formula, the effect will be reduced when a person is lying down, due to the fact that the height of the column will naturally be h = 0 cm, in turning meaning that HSP = 0. That is clearly very plausible, due to the fact that the column of liquid in a catheter that is "lying down" (horizontal) will no longer be squeezed out the end under the force of its own weight. In other words: the ventricle connected to the abdomen will no longer be at risk of being sucked dry either. In such a situation, there will no longer be any "siphoning effect".
It must again be emphasised that this physical effect will ALWAYS occur and is UNAVOIDABLE when the patient stands. When this happens, a huge suction force of 20 - 60 cmH2O will be produced, with the severity depending on the height of the patient or the distance between the ventricle and the diaphragm. The symptoms described above, such as slit ventricle syndrome and the movement of entire areas of the brain can be caused by this alone. Even this purely physical explanation makes it clear, that unlike underdrainage, the very similar symptoms caused by overdrainage ONLY occur when the patient is standing up, and will subside again relatively quickly once the patient lies down. This provides a good diagnostic criterion for when attempting to distinguish one of these phenomena from the other.
Still, low intraventricular pressure is not the primary issue when it comes to overdrainage. Rather, the primary issue is the fact that the expansion of the brain tissue can lead to the formation of hygromas and haematomata (i.e. cavities filled with liquid and blood) particularly when this occurs between the surface of the brain and the skull. The brain is firmly attached to the skull via the intricately-structured arachnoid mater and dura mater, which it usually cannot be detached from. If it is sucked, away from the cranial wall, with too much force these delicate membranes and the small bridging veins running through them will be the first things to rip and start bleeding. However, large venous sinuses in the dura or arteries can also be damaged. Overdrainage almost exclusively causes subdural haematomata (SDH). There is even the possibility that these may become chronic. Laboratory experiments have shown how a typical level of hydrostatic suction in the ventricles of around 30 cmH2O can subject the external membranes of the brain to forces equivalent to several hundred grams in weight. The scenario described above makes it clear how haematomata caused by overdrainage can become chronic (chronic subdural haematomata or cSDH).
Overdrainage causes another, equally serious problem known as "slit ventricle syndrome". The contraction of the ventricle caused by the suction closing the small openings in the ventricular catheter (VC). The CRF can no longer be discharged and the ventricles begin to expand again. If the suction then starts again, the process will go back to the beginning. These periodic contractions and expansions of the ventricle, accompanied by high levels of tension, are, by themselves, enough cause tissue damage. If the tissue continues to grow into the small openings of the catheter, this can also lead to tears in the tissue and minor bleeds when the ventricle then expands. The interior (lining) of the ventricle, the ependyma, becomes scarred and the ventricle solidifies. This is one reason why the size of the ventricle is not a good indicator of the existing pressure ratios.
Lumbo-peritoneal (LP) shunts seem to have become increasingly popular in the last few years, as they make it possible to avoid skull and brain injuries. With LP shunts, the spinal cavity is directly linked to the ventricles. The Liquor is drained from between the third and fifth lumbar vertebrae into the peritoneum. Even today, the view that hydrostatic suction does not play a significant role in LP shunts and that the risk of overdrainage is therefore lower, can still be found in the literature (Bloch8, 2012). This assertion is utterly false and misleading. It probably comes from the fact that in LP shunts, the source of the Liquor (the spinal cavity) and drainage site (the peritoneum) are not located on top of one-another: the shunt catheter between the puncture site and abdomen is actually roughly horizontal, meaning that the hydrostatic level appears to be h = 0.

It has been explained previously, in detail, that when the person stands up, the entire Liquor filled spinal cavity, all the way up to the ventricles, is raised up above the abdomen. Indeed, if the cavity is now open because of the shunt, the column of liquid in the spine can drain into the abdomen, creating the same hydrostatic suction in the ventricles (the height, "h") as would occur in a ventriculo-peritoneal shunt. Given that the spinal cavity is significantly wider than the narrow peritoneal catheter and produces a greater flow of liquid, the overdrainage actually occurs significantly more quickly. In a recent literature review (Miyake9, 2016) a similar misleading remark is made, stating the siphon effect is negligible in LP shunts. However this should be viewed in a distinct way. It is true that in the case of the LP valve, which, of course, is at the same height as the abdomen, not very much suction comes from the abdomen (i.e. from below). Nevertheless, because of this, the entire force of the hydrostatic pressure in the spinal cavity weighs down upon it from above. In the case of a VP shunt, the valve is at the same height as the ventricle and all of the hydrostatic suction comes from below, whereas there is only a relatively low amount of IVP to exert an effect from above. Still, when it comes to a standard differential pressure valve, the only thing that is relevant is the sum of both the amount of pressure and the amount of suction. Therefore, in both cases, the outcome in relation to overdrainage remains the same.
Aside from the existing evidence and considerations of plausibility, it has not been possible to definitively establish whether a specific type of valve is better or worse, even in the last decade. In this area, scientific knowledge, which normally advances at high speed, appears to be progressing at a snail's pace. There are many very important reasons for this. One such reason is clearly the fact that human physiology is highly complex and that it often takes a huge amount of effort to collect reliable statistical data. As a result, it will be necessary to leave sufficient space in a later section for a nuanced weighing-up of the advantages and disadvantages posed by the different types of hydrostatic implants on the market.
Nevertheless, even at this stage, it can and should be pointed out that the effectiveness of gravitational valves in preventing overdrainage has been well-documented in the literature. This applies to every age group. The following list of recent studies only represents a sample of such literature: Al-Hakim10, 2018; Gebert11, 2016; Xinxing12, 2015, Suchorska13, 2015; Kehler14, 2015; Malem7, 2014; Thomale15, 2013; Lemcke16, 2013; Gebert17, 2013; Freimann18, 2013.
One finding of the SVASONA study (Lemcke16, 2013), which is well known amongst neurosurgeons, was that overdrainage can be prevented in one in three patients by using gravitational valves. The rate of overdrainage "in the control group" (i.e. the group who did not have any gravitational valves implanted) of this study was significantly higher than in other studies (Sundstrom19, 2017). However, the corresponding rate in the treatment group (who were implanted with gravitational valves) was also remarkably low. Alfred Aschoff, a hydrocephalus expert who is well known among neurosurgeons, has now noted, in his most-recently published textbook chapter on shunt valves, that this applies to many groups treated with gravitational valves (Aschoff20, 2019).
In this comprehensive text, Aschoff also superimposed the so-called "survival curves" (also known as “Kaplan-Meier curves") on top of one another, so that direct comparisons could be made. "Survival curves" are widely used in shunt therapy due to the fact that they provide a graphic representation (in percentage terms) of how many of the valves that were originally planted have "survived" (i.e. have continued to work) after a certain period of time. The valves not included in the survival curves were those subject to revision, i.e. those that were removed due to a malfunction or complication. Here too, the aforementioned problem of overdrainage is an important and sometimes irreparable complication, which can therefore shorten the service life of the valve. Other important reasons for shunt failure include infections, blockages and mechanical malfunctions, such as a valve breaking or a catheter being torn. In the survival curves compared by Aschoff, all the curves at the top are from studies using gravitational valves. Unlike standard differential valves, only 50-60% of which continued to operate without any malfunctions after a period of two years, around 80% of the gravitational valves were still in place following the same period of time.
The superiority of gravitational valves, as inferred by Aschoff, has even made its way into the official guidelines for the "Diagnostik und Therapie des Normaldruckhydrozephalus" [Diagnosis and Treatment of Normal Pressure Hydrocephalus], which is published periodically by the German Neurological society:
www.awmf.org/uploads/tx_szleitlinien/030-063_S1_Normaldruckhydrozephalus_2018-03.pdf
It is stated, under the heading "Important Recommendations", that,
"If a shunt is implanted to treat idiopathic normal pressure hydrocephalus, a gravitational valve should be used. Differential pressure valves, especially those with in which the valve opening pressure cannot be adjusted, lead to complications relating to overdrainage significantly more often under the same clinical conditions".
Bibliography
1) 2007, Ritz R., Roser F., Morgalla M., Dietz K., Tatagiba M. and Will B. E.: "Do antibiotic-impregnated shunts in hydrocephalus therapy reduce the risk of infection? An observational study in 258 patients",
BMC Infect Dis 7: 38,
Abstr.: BACKGROUND: Shunt infection in hydrocephalus patients is a severe, even life-threatening complication. Antibiotic-impregnated shunts (AIS) have been developed in an attempt to reduce rate of shunt infection. The study was performed to analyze if AIS can diminish the rate of shunt infection. The pathogenic nature of shunt infection in patients with AIS systems and those without antibiotic impregnated shunts (non-AIS) was compared. METHODS: Over a period of 24 months in the Department of Neurosurgery at University Hospital of Tubingen shunt surgery was performed in 258 patients. In 86 patients AIS systems were implanted. Shunt catheters were commercially impregnated with clindamycin and rifampicin. Analysis of the clinical data included sex, age, classification of hydrocephalus, shunt types and risk factors for shunt infection [age (< 1 year and > 80 years), prematurely born patients, external ventricular drainage, former shunt infection, former systemic infection, disturbance of consciousness, former radiation-/chemotherapy]. Infection rates and underlying bacterial pathogens of patients with AIS were compared to patients with implanted non-AIS systems (172 patients). RESULTS: AIS and non-AIS patients did not differ in sex, etiology of hydrocephalus and the shunt type. In the AIS group 72 out of 86 patients had at least one risk factor (83.7 %), compared to 126 patients in the non-AIS group (73.3 %). There was no significant difference between the two groups (p = 0.0629; Fisher's exact test). In patients with no risk factors, only one patient with non-AIS suffered from shunt infection. In patients with one or more risk factors the rate for shunt infection was 7.14 % in patients with non-AIS and 6.94 % in patients with AIS. Former shunt infection (p = 0.0124) was related to higher risk for shunt infection. The use of AIS had therefore no significant advantage (p = 0.8611; multiple logistic regression). Significantly related to a shunt infection was the number of shunt surgeries. 190 interventions in the AIS group (2.21 interventions per patient) and 408 in the non-AIS group (2.37 interventions per patient) had been performed (p = 0.3063; Wilcoxon). There was no shunt infection in the group of patients on whom only one shunt surgery was performed. In patients with at least two shunt surgeries the infection rate was 9%. The infection rate in AIS patients was 5/52 (9.6 %) and in the non-AIS 10/114 (8.77 %), (p = 1.0; Fisher's exact test). Staphylococcus epidermidis was the most frequent pathogen for shunt infection. Fourteen out of 15 infections occurred within the first 6 months of surgery. The most frequent pathogen for shunt infection was S. epidermidis. No toxic or allergic complications were seen using the AIS shunt systems. The presented data show a remarkably low infection rate of 5.8 % in the non-AIS group compared to other studies which demonstrated a significant decrease in the infection rate by AIS. CONCLUSION: AIS did not significantly reduce shunt infection in hydrocephalus patients in the presented study. In the AIS group three patients suffered from shunt infections caused by skin ulceration or neurosurgical procedures with exposure of the cerebrospinal liquor after shunt implantation. AIS was not developed to prevent infection in such cases, therefore an advantage of AIS can not be excluded. In view of the presented data and the small number of reported studies a prospective randomized multicenter study is required.
2) 2015, Feb, Parker S. L., McGirt M. J., Murphy J. A., Megerian J. T., Stout M. and Engelhart L.: "Comparative effectiveness of antibiotic-impregnated shunt catheters in the treatment of adult and pediatric hydrocephalus: analysis of 12,589 consecutive cases from 287 US hospital systems",
J Neurosurg 122(2): 443-448,
Abstr.: OBJECT: The real-world effectiveness of antibiotic-impregnated shunt catheters to reduce the incidence of shunt infections is still debated. The literature to date consists mostly of small, single-institution studies. The aim of this study was to assess the incidence of infection for antibiotic-impregnated catheters (AICs) versus standard shunt catheters in a large nationwide administrative database. METHODS: The authors retrospectively reviewed hospital discharge and billing records from the Premier Perspective Database from April 2003 to July 2009 to identify all adult and pediatric patients undergoing de novo ventricular shunt placement. The primary end point was the incidence of shunt infection within 1 year of implantation. Multivariate logistical regression was performed to determine factors associated with increased incidence of infection. RESULTS: A total of 10,819 adult (AIC, 963; standard catheter, 9856) and 1770 pediatric (AIC, 229; standard catheter, 1541) patients underwent ventricular shunt placement in 287 US hospitals. Overall, the incidence of infection was 3.5% in adults (n=380) and 6.6% in pediatric patients (n=116). AICs were associated with significant reduction in infection for both adult (2.2% vs 3.6%, p=0.02) and pediatric (2.6% vs 7.1%, p<0.01) patients. AIC use was associated with reduced infection regardless of hospital size, annual shunt volume, hospital location, or patient risk factors and remained associated with a reduced infection in multivariate analysis for both adult (p=0.02) and pediatric (p=0.02) patients. CONCLUSIONS: The use of antibiotic-impregnated shunt catheters was associated with a reduction in shunt infections for both adult and pediatric patients. This provides further support that AICs may represent a reliable means of reducing shunt infections for both adult and pediatric patients.
3) 2004, Oct, Bergsneider M., Yang I., Hu X., McArthur D. L., Cook S. W. and Boscardin W. J.: "Relationship between valve opening pressure, body position, and intracranial pressure in normal pressure hydrocephalus: paradigm for selection of programmable valve pressure setting",
Neurosurgery 55(4): 851-858; discussion 858-859,
Abstr.:OBJECTIVE: There is little scientific basis for guidance in selecting the optimal valve for the treatment of normal pressure hydrocephalus. The aim of this study was to determine the programmable valve opening pressure setting that would result in a slight reduction in intracranial pressure (ICP) after a ventriculoperitoneal shunt is implanted. We also assessed whether shunt-induced ICP could be predicted on the basis of a simple hydrodynamic equation. METHODS: In this prospective study of 11 patients with normal pressure hydrocephalus, ICP was measured before and after implantation of a shunt incorporating a programmable valve without an antisiphon device. Pressure measurements, including intraperitoneal pressure, were recorded at body angles ranging from 0 to 55 degrees and at valve settings ranging from 30 to 200 mm H(2)O. Measured ICP values were compared with values computed using a simple hydrodynamic equation. RESULTS: Even at a valve setting greater than the mean baseline ICP (200 mm H(2)O), the supine ICP was significantly lower than the baseline value (baseline ICP, 164 +/- 64 mm H(2)O; postoperative ICP, 125 +/- 69 mm H(2)O, P = 0.04). Valve pressure did not equate 1:1 with the measured postoperative ICP. Comprehensive ICP measurements at upright body positions demonstrated a stepwise reduction in ICP rather than a precipitous decline as a result of so-called siphoning. CONCLUSION: This study indicates that very high valve opening pressure settings may be optimal for the initial treatment of normal pressure hydrocephalus. The relationship between ICP and opening pressure valves is linear but not predicted by simple hydrodynamics.
4) 2018, Feb, Pedersen S. H., Lilja-Cyron A., Andresen M. and Juhler M.: "The Relationship Between Intracranial Pressure and Age-Chasing Age-Related Reference Values",
World Neurosurg 110: e119-e123,
Abstr.: BACKGROUND: No true reference values for intracranial pressure (ICP) in humans exist; current values are estimated from measurements in adults who undergo treatment in order to correct ICP. We report ICP values in a "pseudonormal" group of children and adults to examine if age affects ICP. METHODS: We analyzed data from all nonshunted patients undergoing a 24-hour ICP monitoring as part of a diagnostic work-up and included patients with no subsequent suspicion of increased ICP and no need for pressure-relieving treatment with a minimum follow-up period of 3 years. RESULTS: From February 2008 to November 2014, a 24-hour ICP monitoring was performed in 221 patients. Of these patients, 35 (14 children, 21 adults) met the inclusion criteria. Follow-up time to confirm absence of ICP-related disease was 3-9 years. Daytime ICP was 2.8 mmHg +/- 2.2 in children and 1.9 mmHg +/- 4.2 in adults (P = 0.39). Of 35 patients, 32 had higher nighttime ICP. The difference between daytime and nighttime ICP was similar in children (DeltaICP = 5.8 mmHg +/- 4.0, P < 0.0001) and adults (DeltaICP = 6.1 mm Hg +/- 3.3, P < 0.0001). ICP could be described as a decreasing function of age, with an ICP decrement of 0.69 mmHg per decade (P = 0.015). CONCLUSIONS: We found similar differences in daytime and nighttime ICP between children and adults with no ICP-related disease. ICP seems to decrease with age across all ages. This has implications for therapeutic interventions (e.g., shunt valve selection or resistance in external ventricular drainage).
5) 2018, Jan, Antes S., Stadie A., Muller S., Linsler S., Breuskin D. and Oertel J.: "Intracranial Pressure-Guided Shunt Valve Adjustments with the Miethke Sensor Reservoir",
World Neurosurg 109: e642-e650,
Abstr.: BACKGROUND: Telemetric intracranial pressure (ICP) monitoring seems to be a promising therapy-supporting option in shunt-treated patients. Benefits become obvious when headaches are unspecific and clinical symptoms cannot be related to possible overdrainage or underdrainage. In this study, we evaluated a new telemetric device to individually adjust shunt valves according to ICP measurements. METHODS: Between December 2015 and November 2016, 25 patients with suspected suboptimal shunt valve settings underwent insertion of a telemetric ICP sensor (Sensor Reservoir; Christoph Miethke, Potsdam, Germany). Over a 1-year period, a total of 183 telemetric ICP measurements and 85 shunt valve adjustments were carried out. Retrospective statistic analyses focused on valve adjustments, ICP values, and clinical outcomes. RESULTS: ICP-guided valve adjustments positively changed the clinical state in 18 out of 25 patients. Clinical improvement over time was associated with significant changes of the valve settings and ICP values. Interestingly, a therapeutically normalized ICP profile was not automatically associated with clinical improvement. CONCLUSIONS: The Sensor Reservoir is an important and valuable tool for shunt-treated patients suffering from drainage-related problems. The possibility to simultaneously recognize and solve shunt problems represents the decisive advantage. Nevertheless, measurements with the Sensor Reservoir did not allow for the determination of default valve settings or universal target ICP values.
6) 1998, Mar, Boon A. J., Tans J. T., Delwel E. J., Egeler-Peerdeman S. M., Hanlo P. W., Wurzer H. A., Avezaat C. J., de Jong D. A., Gooskens R. H. and Hermans J.: "Dutch Normal-Pressure Hydrocephalus Study: randomized comparison of low- and medium-pressure shunts",
J Neurosurg 88(3): 490-495,
Abstr.: OBJECT: The goal of this prospective study was to compare outcome after placement of a low- or medium-pressure shunt in patients with normal-pressure hydrocephalus (NPH). METHODS: Ninety-six patients with NPH were randomized to receive a low-pressure ventriculoperitoneal shunt (LPV; 40 +/- 10 mm H2O) or medium high-pressure ventriculoperitoneal shunt (MPV; 100 +/- 10 mm H2O). The patients' gait disturbance and dementia were quantified by applying an NPH scale, and their level of disability was evaluated by using the modified Rankin scale (mRS). Patients were examined prior to and 1, 3, 6, 9, and 12 months after surgery. Primary outcome measures were determined by differences between preoperative and last NPH scale scores and mRS grades. The LPV and MPV shunt groups were compared by calculating both the differences between mean improvements and the proportions of patients showing improvement. Intention-to-treat analysis of mRS grades yielded a mean improvement of 1.27 +/- 1.41 for patients with LPV shunts and 0.68 +/- 1.58 for patients with MPV shunts (p = 0.06). Improvement was found in 74% of patients with LPV shunts and in 53% of patients with MPV shunts (p = 0.06) and a marked-to-excellent improvement in 45% of patients with LPV shunts and 28% of patients with MPV shunts (p = 0.12). All outcome measures indicated trends in favor of the LPV shunt group, with only the dementia scale reaching significance. After exclusion of serious events and deaths unrelated to NPH, efficacy analysis showed the advantage of LPV shunts to be diminished. Reduction in ventricular size was also significantly greater for patients in the LPV shunt group (p = 0.009). Subdural effusions occurred in 71% of patients with an LPV shunt and in 34% with an MPV shunt; however, their influence on patient outcome was limited. CONCLUSIONS: Outcome was better for patients who had an LPV shunt than for those with an MPV shunt, although most differences were not statistically significant. The authors advise that patients with NPH be treated with an LPV shunt.
7) 2014, Aug 21, Malem D. N., Shand Smith J. D., Toma A. K., Sethi H., Kitchen N. D. and Watkins L. D.: "An investigation into the clinical impacts of lowering shunt opening pressure in idiopathic normal pressure hydrocephalus: A case series",
Br J Neurosurg: 1-5,
Abstr.: Introduction. Idiopathic normal pressure hydrocephalus (iNPH) is a shunt- reversible syndrome of the elderly. Shunt management is aimed at achieving a balance between clinical improvement and the complications associated with overdrainage. Although clinical improvement occurs at low pressure, these benefits may be negated by the increase in complication rates observed at lower pressures. The addition of gravity-switch devices has been shown to reduce over drainage problems even at a low valve pressure setting. At our centre the Miethke proGAV is used and commonly lowered below 5 cmH2O to gain further clinical improvement. Object. To determine whether lowering the opening pressure to below 5cmH2O using the proGAV valve in iNPH patients results in a) improved clinical features; and b) no significant increase in complication rates. Methods. A retrospective case series of iNPH patients was undertaken with 24 patients who had the proGAV shunt system inserted with an initial opening pressure of 5cmH2O. Exclusion criteria were secondary NPH, shunt system other than proGAV inserted, no valve adjustment to below 5cmH2O and inadequate follow-up. Outcome measures were clinical improvement (gait, cognition and urinary continence) and complications (subdural haematoma, low-pressure symptoms and valve damage). Results. Patients underwent a total of 29 adjustments to below 5cmH2O. The mean valve opening pressure after the first adjustment was 2.5cmH2O and the mean opening pressure after the second adjustment was 1cmH2O. Overall, outcome after adjustment included 26% no change, 48% improvement and 26% deterioration clinically. One patient (4%) suffered traumatic subdural haematoma that resolved with increasing valve pressure to 20cmH2O. There was no valve damage or low-pressure symptoms after adjustment. Conclusion. This study found that lowering the opening pressure of the proGAV shunt system to below 5cmH2O results in clinical improvement and does not significantly increase the complication rate in iNPH patients.
8) 2012, Aug, Bloch O. and McDermott M. W.: "Lumboperitoneal shunts for the treatment of normal pressure hydrocephalus",
J Clin Neurosci 19(8): 1107-1111,
Abstr.: Ventriculoperitoneal shunt placement is the standard of care for idiopathic normal pressure hydrocephalus (iNPH). Studies have reported shunt complication rates up to 38%, with subdural hemorrhage rates as high as 10%. Lumboperitoneal (LP) shunts with horizontal-vertical valves (HVV) are an alternative for cerebrospinal fluid (CSF) diversion that avoids direct cerebral injury and may reduce the risk of overdrainage. Here we reviewed our experience with LP-HVV shunt placement for iNPH. We retrospectively reviewed our 33 patients with LP-HVV shunts inserted for the treatment of iNPH from 1998 to 2009. Patients were evaluated for improvements in gait, urinary function, and dementia after shunt placement. All patients had evidence of ventriculomegaly and a positive response to pre-operative lumbar puncture or extended lumbar drainage. All 33 (100%) patients had pre-operative gait dysfunction, 28 (85%) had incontinence, and 20 (61%) had memory deficits. Mean follow-up time was 19 months. Following shunt placement, 33/33 (100%) patients demonstrated improved gait, 13/28 (46%) had improvement in incontinence, and 11/20 (55%) had improvement in memory. Shunt failures requiring revision occurred in nine patients (27%), with an average time to failure of 11 months. Infections occurred in two patients (6%). There were no neurologic complications, including no hemorrhages. Thus, LP-HVV shunt placement is a safe and effective alternative to ventriculoperitoneal shunting for iNPH, resulting in significant symptomatic improvement with a low risk of overdrainage. It should be considered as an option for the treatment of patients with iNPH who demonstrate clinical improvement following lumbar drainage.
9) 2016, May 15, Miyake H.: "Shunt Devices for the Treatment of Adult Hydrocephalus: Recent Progress and Characteristics",
Neurol Med Chir (Tokyo) 56(5): 274-283,
Abstr.: Various types of shunt valves have been developed during the past 50 years, most of which can be classified into the following categories: (1) fixed differential pressure valves; (2) fixed differential pressure (DP) valves with an antisiphon mechanism; (3) programmable DP valves; (4) programmable DP valves with an antisiphon mechanism; and (5) programmable antisiphon valves. When considering the myriad of possible postoperative condition changes, such as the onset of accidental non-related diseases or trauma in adults, and changes in normal physiological development or anticipation of future shunt removal in children, it has become standard to use the programmable valve as a first choice for cerebrospinal fluid shunting. However, it is still unclear what type of shunt valve is suitable for each individual case. Based on the results of SINPHONI and more recently SINPHONI 2 trials, the programmable DP valve is recommended as the first line shunt valve. The programmable DP valve with an antisiphon mechanism is thought to be beneficial for tall, slender patients, who have a tendency for easily developing complications of overdrainage, however, this type of valve must be used cautiously in obese patients because of the increased risk of underdrainage. Although the current evidence is still insufficient, the programmable antisiphon valve, which costs the same as the programmable DP valve, is also thought to be the first line shunt valve. The quick reference table is applicable for most shunt valves, and for patients with either the ventriculoperitoneal or the lumboperitoneal shunt.
10) 2018, Jul, Al-Hakim S., Schaumann A., Schneider J., Schulz M. and Thomale U. W.: "Experience in shunt management on revision free survival in infants with myelomeningocele",
Childs Nerv Syst 34(7): 1375-1382,
Abstr.: OBJECTIVE: Depending on the etiology of hydrocephalus in childhood, the shunt therapy still remains challenging due to frequent shunt complications leading to possible revisions such as shunt infection or shunt malfunction. In myelomeningocele (MMC) patients who often require shunt therapy, higher revisions rates were reported. In a single-center retrospective study, experiences on shunt regimen on hydrocephalus associated with MMC are presented. METHODS: Data of 160 infant hydrocephalus cases younger than 1 year of age at the time of implantation were retrospectively reviewed from the hospital database. These patients received an adjustable differential pressure valve with gravitational unit and antibiotic impregnated catheters as a primary or secondary implant during the time period of April 2007 to July 2015. The subgroup of infants cases with MMC (n = 44; age 50.6 +/- 80.6 days) were compared to the remaining cohort of other hydrocephalus etiology (control group). The shunt and valve revision free survival rates were recorded until July 2017. RESULTS: During the mean follow-up of 48.7 +/- 19.2 (7-114) months, the shunt revision free survival was 87% at 1 year and 49% at 60 months in the MMC cohort. The control group showed a shunt survival rate of 68% at 1 year and 39% at 60 months. Similarly, the valve revision free survival rate showed a significant higher rate of 92% at 1 year and 69% at 60 months in the MMC group compared to the control group (75% at 1 year and 51% at 60 months; p < 0.05). During the entire follow-up period, 37% of the MMC infants underwent a revision operation in contrast to the control group of 40%. CONCLUSION: The presented shunt strategy showed improved revision free survival rates in infants with a MMC-related hydrocephalus in comparison to other etiologies of hydrocephalus in infants, which might relate to infection prophylaxis and high drainage resistance integrated in the shunt system.
11) 2016, May, Gebert A. F., Schulz M., Schwarz K. and Thomale U. W.: "Long-term survival rates of gravity-assisted, adjustable differential pressure valves in infants with hydrocephalus",
J Neurosurg Pediatr 17(5): 544-551,
Abstr.: OBJECTIVE The use of adjustable differential pressure valves with gravity-assisted units in shunt therapy of children with hydrocephalus was reported to be feasible and promising as a way to avoid chronic overdrainage. In this single-center study, the authors' experiences in infants, who have higher rates of shunt complications, are presented. METHODS All data were collected from a cohort of infants (93 patients [37 girls and 56 boys], less than 1 year of age [mean age 4.1 +/- 3.1 months]) who received their first adjustable pressure hydrocephalus shunt as either a primary or secondary implant between May 2007 and April 2012. Rates of valve and shunt failure were recorded for a total of 85 months until the end of the observation period in May 2014. RESULTS During a follow-up of 54.2 +/- 15.9 months (range 26-85 months), the Kaplan-Meier rate of shunt survival was 69.2% at 1 year and 34.1% at 85 months; the Kaplan-Meier rate of valve survival was 77.8% at 1 year and 56% at 85 months. Survival rates of the shunt were significantly inferior if the patients had previous shunt surgery. During follow-up, 44 valves were exchanged in cases of infection (n = 19), occlusion (n = 14), dysfunction of the adjustment unit (n = 10), or to change the gravitational unit (n = 1). CONCLUSIONS Although a higher shunt complication rate is observed in infant populations compared with older children, reasonable survival rates demonstrate the feasibility of using this sophisticated valve technology. The gravitational unit of this valve is well tolerated and its adjustability offers the flexible application of opening pressure in an unpredictable cohort of patients. This may adequately address overdrainage-related complications from early in treatment.
12) 2015, Aug, Xinxing L., Hongyu D. and Yunhui L.: "Using individualized opening pressure to determine the optimal setting of an adjustable proGAV shunt in treatment of hydrocephalus in infants",
Childs Nerv Syst 31(8): 1267-1271,
Abstr.: INTRODUCTION: The aim of the study was to describe the authors' experiences with the adjustable gravitational proGAV shunt system in treating infants' hydrocephalus. PATIENTS AND METHODS: The proGAV was implanted in 29 infants (11 boys and 18 girls, median age 9 months) with hydrocephalus of various origins between January 2010 and June 2014. The opening pressure was chosen based on the intraventricular pressure which was measured during operation, and a gravitational unit with a fixed opening pressure (15 cm H2O) was selected. RESULT: Regular clinical follow up ranged from 6 to 36 months, with a mean follow-up time of 15.7 +/- 7.9 months. Five of these patients (17.2%) underwent shunt revision because of malfunction, including two infections (6.9%), two shunt exposed (6.9%), and one proximal catheter occlusion (3.4%). For readjustment in 10 infants, the opening pressure was changed at least once during the follow-up period. CONCLUSION: The proGAV is efficacious in the treatment of hydrocephalus in infants. This individual selection of the opening pressures makes it probable that a better match of chosen opening pressure and actual hydraulic requirements of the patient might occur.
13) 2015, Apr, Suchorska B., Kunz M., Schniepp R., Jahn K., Goetz C., Tonn J. C. and Peraud A.: "Optimized surgical treatment for normal pressure hydrocephalus: comparison between gravitational and differential pressure valves",
Acta Neurochir (Wien) 157(4): 703-709,
Abstr.: OBJECTIVES: In idiopathic normal pressure hydrocephalus (NPH) ventriculoperitoneal (VP) shunt insertion is the method of choice to improve cardinal symptoms such as gait disturbance, urge incontinence and/or dementia. With reduced compliance, the brain of the elderly is prone for overdrainage complications. This was especially true with the use of differential pressure valve implantation. The present study compares clinical outcome and complication rates after VP shunt insertion with differential pressure valves in the early years and gravitational valves since 2005. METHODS: The authors reviewed patients treated at our institution for NPH since 1995. Differential pressure valves were solely used in the initial years, while the treatment regimen changed to gravitational valves in 2005. Clinical improvement/surgical success rates as well as complications were compared between the two groups. RESULTS: Eighty-nine patients were enrolled for the present study. Mean age at the time of surgery was 73.5 +/- 6.3 years. Male patients predominated with 73, compared with 16 female patients. Median follow-up time was 28 +/- 26 months. Date of last follow-up was 1st October 2013. Forty-nine patients received a gravitational valve, while 40 were treated with differential pressure valves. In the gravitational group a significant improvement was observed after shunt insertion for gait disorder, cognitive impairment and urge incontinence (p < 0.0001, resp. p = 0.004), while a significant change in the differential pressure group was only seen for gait disorder (p = 0.03) but not for cognition or urinary incontinency (p > 0.05). The risk of hygroma as a sign of shunt overdrainage requiring surgical intervention was significantly higher in the differential pressure group (5 versus 0 in the gravitational group). CONCLUSIONS: Patients with NPH treated with gravitational valves in the present cohort showed a more profound improvement in their initial symptoms, including gait disorder, cognitive impairment and urinary incontinency without the risk of overdrainage complications requiring surgical intervention when compared with patients who received differential pressure valves in previous years.
14) 2015, Oct, Kehler U., Kiefer M., Eymann R., Wagner W., Tschan C. A., Langer N., Rohde V., Ludwig H. C., Gliemroth J., Meier U., Lemcke J., Thomale U. W., Fritsch M., Krauss J. K., Mirzayan M. J., Schuhmann M. and Huthmann A.: "PROSAIKA: a prospective multicenter registry with the first programmable gravitational device for hydrocephalus shunting",
Clin Neurol Neurosurg 137: 132-136,
Abstr.: OBJECTIVE: Cerebrospinal fluid (CSF) overdrainage is a major problem in shunt therapy for hydrocephalus. The adjustable gravitational valve proSA allows for the first time a targeted compensation for overdrainage in the upright position without interfering with the differential pressure valve. To evaluate benefit, safety and reliability, the multicenter prospective registry PROSAIKA was conducted in 10 German neurosurgical centers. METHODS: Between March 2009 and July 2010, 120 hydrocephalic patients undergoing first time shunt implantation or shunt revision using proSA entered the study. 93 patients completed the 12 months follow-up. RESULTS: Hydrocephalus symptoms were improved in 86%, unchanged in 9% and deteriorated in 3%. In 51%, the proSA opening pressure was readjusted one or several times to treat suspected suboptimal shunt function, this resulted in clinical improvement in 55%, no change in 25%, and deterioration in 20% of these patients. The 1 year censored proSA shunt survival rate was 89%. Device related shunt failure was seen in two cases. CONCLUSIONS: This is the first clinical report on the implantation of the adjustable gravitational valve proSA with a follow-up of 12 months in a substantial number of patients. Irrespective of different hydrocephalus etiologies and indications for shunt surgery, the overall results after 12 months were very satisfying. The high frequency of valve readjustments underlines the fact that preoperative selection of the appropriate valve opening pressure is difficult. The low number of revisions and complications compared to other valves proves that proSA implantation adds no further risk; this valve is reliable, helpful and safe.
15) 2013, Mar, Thomale U. W., Gebert A. F., Haberl H. and Schulz M.: "Shunt survival rates by using the adjustable differential pressure valve combined with a gravitational unit (proGAV) in pediatric neurosurgery",
Childs Nerv Syst 29(3): 425-431,
Abstr.: OBJECTIVES: Overdrainage is a chronic complication in shunted pediatric patients with hydrocephalus. The use of adjustability of differential pressure (DP) valves in combination with antisiphoning devices may help to overcome this sequela and may diminish the rate of possible shunt failures. The purpose of this retrospective study is to report our experience on shunt survival and infection rate with an adjustable DP valve with integrated gravitational unit in pediatric hydrocephalus. METHODS: The proGAV consists of an adjustable differential pressure (DP) valve and a gravitational unit. During the time period of July 2004 and December 2009, a total of 237 adjustable gravitational valves were used in 203 children (age, 6.5 +/- 6.54; 0-27 years). In the follow-up period, valve and shunt failures as well as rate of infection were recorded. RESULTS: Within the average follow-up time of 21.9 +/- 10.3 months (range, 6-72 months), the valve survival rate was 83.8 %. The overall shunt survival rate including all necessary revisions was 64.3 %. Looking at the group of infants (<1 year of age) within the cohort, the valve survival rate was 77.3 % and the shunt survival rate was 60.9 %. The overall infection rate was 4.6 %. CONCLUSION: In a concept of avoiding chronic overdrainage by using the proGAV in hydrocephalic children, we observed a good rate of valve and shunt survival. Compared to previous reported series, we experienced the proGAV as a reliable tool for the treatment of pediatric hydrocephalus.
16) 2013, Aug, Lemcke J., Meier U., Muller C., Fritsch M. J., Kehler U., Langer N., Kiefer M., Eymann R., Schuhmann M. U., Speil A., Weber F., Remenez V., Rohde V., Ludwig H. C. and Stengel D.: "Safety and efficacy of gravitational shunt valves in patients with idiopathic normal pressure hydrocephalus: a pragmatic, randomised, open label, multicentre trial (SVASONA)",
J Neurol Neurosurg Psychiatry 84(8): 850-857,
Abstr.: OBJECTIVES: To investigate whether gravitational valves reduce the risk of overdrainage complications compared with programmable valves in ventriculoperitoneal (VP) shunt surgery for idiopathic normal pressure hydrocephalus (iNPH). BACKGROUND: Patients with iNPH may benefit from VP shunting but are prone to overdrainage complications during posture changes. Gravitational valves with tantalum balls are considered to reduce the risk of overdrainage but their clinical effectiveness is unclear. METHODS: We conducted a pragmatic, randomised, multicentre trial comparing gravitational with non-gravitational programmable valves in patients with iNPH eligible for VP shunting. The primary endpoint was any clinical or radiological sign (headache, nausea, vomiting, subdural effusion or slit ventricle) of overdrainage 6 months after randomisation. We also assessed disease specific instruments (Black and Kiefer Scale) and Physical and Mental Component Scores of the Short Form 12 (SF-12) generic health questionnaire. RESULTS: We enrolled 145 patients (mean (SD) age 71.9 (6.9) years), 137 of whom were available for endpoint analysis. After 6 months, 29 patients in the standard and five patients in the gravitational shunt group developed overdrainage (risk difference -36%, 95% CI -49% to -23%; p<0.001). This difference exceeded predetermined stopping rules and resulted in premature discontinuation of patient recruitment. Disease specific outcome scales did not differ between the groups although there was a significant advantage of the gravitational device in the SF-12 Mental Component Scores at the 6 and 12 month visits. CONCLUSIONS: Implanting a gravitational rather than another type of valve will avoid one additional overdrainage complication in about every third patient undergoing VP shunting for iNPH.
17) 2013, Nov, Gebert A. F., Schulz M., Haberl H. and Thomale U. W.: "Adjustments in gravitational valves for the treatment of childhood hydrocephalus-a retrospective survey",
Childs Nerv Syst 29(11): 2019-2025,
Abstr.: OBJECTIVE: Debatable disadvantages of preset differential pressure valves in treating pediatric hydrocephalus are the inability to treat over- and underdrainage after implantation without further surgery. This survey aims to retrospectively determine whether adjustments were performed and effectively used for the individual adaptation of CSF drainage from the shunted patients' and families' perspective. METHODS: In a series of 132 consecutive patients (59girls; 73boys, 0-29 years), families, caretakers, or the patients themselves were interviewed about their experiences after using the proGAV (Miethke-Aesculap, Germany) within a CSF-diverting shunt system. Thereby, the necessity and amounts of adjustments were evaluated. The subjective experiences of the adjustment process as well as the subsequent surgical interventions were documented with a follow-up period of 25.6 +/- 9 months. RESULTS: In 87.9 % of the cases, clinical symptoms improved subjectively after valve implantation. A total of 103 adjustments in 69 patients were performed. In 30 % of patients, more than one readjustment was done. As subjective experience, the adjustment process was described by 85 % of patients as painless or merely uncomfortable. Symptoms improved in 91 % in connection to a new pressure setting. During the entire follow-up period, 61 % of all patients remained free of surgery. CONCLUSION: The recently used adjustable valves provide good clinical results and seem to lead to satisfactory treatment from the perspective of the affected patients or caretakers. Although a mechanical manipulation of the skin is necessary, the mechanism of the integrated adjustment unit was mostly well tolerated and allows for a noninvasive and MRI stable treatment of over- and underdrainage.
18) 2013, Jul 4, Freimann F. B., Vajkoczy P. and Sprung C.: "Patients benefit from low-pressure settings enabled by gravitational valves in normal pressure hydrocephalus",
Clin Neurol Neurosurg 115(10): 1982-1986,
Abstr.: OBJECTIVE: The ability of siphon regulatory devices to improve overdrainage control despite low-pressure settings of the valve for the horizontal body position has been described previously. We aim to provide a systematic investigation on the ability of gravitational units as siphon regulatory devices to improve clinical outcome in shunt therapy. METHODS: We analyzed retrospectively postoperative complications, type and frequency of valve adjustments and the clinical outcome using Black's outcome scale at different time points of all iNPH patients operated in our center between January 2007 and December 2010. They had received either a proGAV(R) valve with an integrated gravitational unit, or a programmable Codman Hakim(R) valve without an integrated siphon regulatory device. RESULTS: The postoperative course of 55 proGAV(R) and 45 programmable Codman Hakim(R) patients was analyzed. The latest documented valve setting of the proGAV(R) group and Codman Hakim(R) group was median 50mm H2O and 120mm H2O, respectively. Overdrainage occurred among both groups in 20% of the patients, while surgical intervention for overdrainage-related complications was seen to be necessary only in 7% of the cases in the Codman Hakim(R) group. Clinical outcome differed in an increasing manner over the observation period (median 4 points after 3, 12 months and at final presentation in the proGAV(R); median 4 points after 3 and 12 months and 3 points at final presentation in Codman Hakim(R) group (p=0.001)). CONCLUSION: Adjustable and gravity-assisted valves like the proGAV(R) improve overdrainage control and enable thus low-pressure settings for the horizontal body position. We observed an improved and more sustainable functional outcome for iNPH patients with an adjustable and gravity-assisted valve compared to iNPH patients without an integrated siphon regulatory device.
19) 2017, Oct 27, Sundstrom N., Lagebrant M., Eklund A., Koskinen L. D. and Malm J.: "Subdural hematomas in 1846 patients with shunted idiopathic normal pressure hydrocephalus: treatment and long-term survival",
J Neurosurg: 1-8,
Abstr.: OBJECTIVE Subdural hematoma (SDH) is the most common serious adverse event in patients with shunts. Adjustable shunts are used with increasing frequency and make it possible to noninvasively treat postoperative SDH. The objective of this study was to describe the prevalence and treatment preferences of SDHs, based on fixed or adjustable shunt valves, in a national cohort of patients with shunted idiopathic normal pressure hydrocephalus (iNPH), as well as to evaluate the effect of SDH and treatment on long-term survival. METHODS Patients with iNPH who received a CSF shunt in Sweden from 2004 to 2015 were included in a prospective quality registry (n = 1846) and followed regarding SDH, its treatment, and mortality. The treatment of SDH was categorized into surgery, opening pressure adjustments, or no treatment. RESULTS During the study period, the proportion of adjustable shunts increased from 75% to 95%. Ten percent (n = 184) of the patients developed an SDH. In 103 patients, treatment was solely opening pressure adjustment. Surgical treatment was used in 66 cases (36%), and 15 (8%) received no treatment. In patients with fixed shunt valves, 90% (n = 17) of SDHs were treated surgically compared with 30% (n = 49) in patients with adjustable shunts (p < 0.001). There was no difference in long-term patient survival between the SDH and non-SDH groups or between different treatments. CONCLUSIONS SDH remains a common complication after shunt surgery, but adjustable shunts reduced the need for surgical interventions. SDH and treatment did not significantly affect survival in this patient group, thus the noninvasive treatment offered by adjustable shunts considerably reduces the level of severity for this common adverse event.
20) 2019, Aschoff A: "In-Depth View: Functional Characteristics of CSF Shunt Devices (Pros and Cons)",
In “Textbook of Pediatric Neurosurgery” (Editor: Di Rocco)