The Innovative Leap to Telemetric Pressure Sensors
Prologue
show moreThe Innovative Leap to Telemetric Pressure Sensors
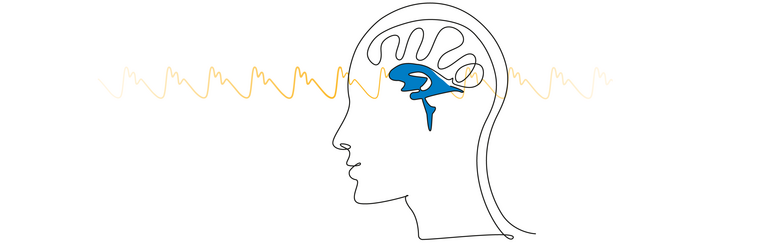
Because these sensors must be physically connected to external readout units for measurement, they can only be used in medical facilities and under controlled conditions. They also limit patient mobility and pose a risk of infection.5-7 Since this risk of infection correlates with the duration of the measuring, these conventional ICP sensors are not suitable for monitoring over several days or weeks.4
However, various conditions necessitate repeated monitoring of intracranial pressure over several years to ensure satisfactory therapeutic outcomes.8 For example, ICP-guided valve adjustment for the therapy of nonspecific and potentially dangerous headaches has proven to be a useful tool in hydrocephalus management.9 Most importantly, long-term monitoring of ICP provides a quantitative method for diagnosis and therapy support that is clearly superior to vague interpretation of symptoms (e.g., headache, nausea).5 Patients undergoing repeated conventional ICP monitoring currently require multiple surgical procedures.
In addition to the outlined risks, conventional ICP sensors have several other drawbacks. Many of these sensors require complex initial preparation and calibration by medical personnel prior to implantation in order to achieve sufficient accuracy over the implantation period.10 Current ICP monitors also exhibit significant baseline drift due to aging of the sensor technology, among other factors.7 Along with spontaneous strong baseline shifts (> 10–20 mmHg) due to sensitivity to electrostatic discharge and temperature fluctuations, this leads to increasing inaccuracies as the measurement period draws on.11-13 Lack of means to verify pressure readings can lead to treatment decisions based on incorrect ICP values.11 Replacement of conventional ICP sensors may be necessary as early as five days after implantation due to observed drift.14 Moreover, up to 13% of patients have reported accidental dislocation due to patient movement, breakage, or malfunction, which also requires replacement of the conventional ICP sensor.15 Some of the conventional ICP sensors contain ferromagnetic components; therefore, the respective patients cannot have MRI scans.16
These are some additions to the detailed discussion of the technical requirements for ICP sensors and the limitations of conventional systems. Collectively, they provide a comprehensive set of market and patient requirements for sensors measuring intracranial pressures: ICP sensors should be accurate, reliable, stable over time with low drift and a sampling rate > 25 Hz as well as high data transmission quality. They should also be as small and easy to use as possible and cause few infections and hemorrhagic complications over a long lifetime.
With that in mind, telemetric sensors for measuring intracranial pressure represent a promising path towards the future.
The testing of shunt function is highly relevant: Suspected malfunction or obstruction remains one of the most common reasons for shunt revision.19-21
In fact, shunt-related complications can occur in one in four patients19, making efficient shunt function checks extremely important. Shunt revisions massively increase the burden on patients and nurses and further encroach on the already limited availability of neurosurgeons. The suspicions are not always confirmed after surgical removal of the shunt, since subpar or impaired shunt function often cannot be diagnosed with certainty using conventional methods such as CT, MRI, or pumping.22-27
CT is also associated with detrimental increased radiation exposure.28 It is difficult to reliably determine what proportion of revisions were actually retrospectively justified due to acute shunt or catheter dysfunction. A study suitable for this purpose might have to classify the revision as unnecessary or redundant after a thorough examination of the shunt. The analysis of our complaints database, which has been comprehensively maintained over a decade, indeed shows that the reported issues (such as blockage) cannot be proven for the majority of shunt components with reported issues. This applies to the more voluminous shunt components, such as the various valves and reservoirs. Unfortunately, clogged or ruptured catheters are rarely returned, rendering it difficult to make valid quantitative statements. However, for patients as well as for treating physicians, it is of highest priority to avoid unnecessary procedures. Furthermore, the reduction of unnecessary costs and the economical use of resources have always been key elements in healthcare. Consequently, non-invasive, non-debilitating, yet safe shunt function checks, like the ones offered by the M.scio, can be extraordinarily important.
The implant design of the M.scio is based on the design of typical components of the vast majority of shunts, such as burrhole or inline reservoirs. As a result, the M.scio does not require any additional surgical steps compared to a standard shunt implantation. The included pressure sensor is miniaturized and integrated into the implant in such a way that it does not affect the flow characteristics of the shunt in any way. Since the measuring electronics obtain the necessary power for measurements from the electromagnetic field of the antenna of the corresponding reader unit set, the M.scio also does not require its own battery; therefore, unlike in other active implants, the batteries never have to be replaced. Together with the complete hermetic encapsulation of the measurement electronics in a robust titanium casing, this enables a theoretically unlimited implantation period of the M.scio.
Note
For regulatory reasons, the specification of a minimum service life is required. The specified value for the M.scio is five years. There are, however, no technical reasons or clinical indications that would limit proper functioning of the M.scio beyond this formally defined lifetime.
As shown over at least four years of use, the almost complete absence of drift in measured values, with deviations of less than 2 mmHg in four years, allows reliant functionality even after a long time.
Even in the unlikely event of a total failure of its pressure measurement function, the M.scio does not have to be revised; instead, it continues to perform the function of a simple reservoir, a prechamber, a deflector, or a connector.
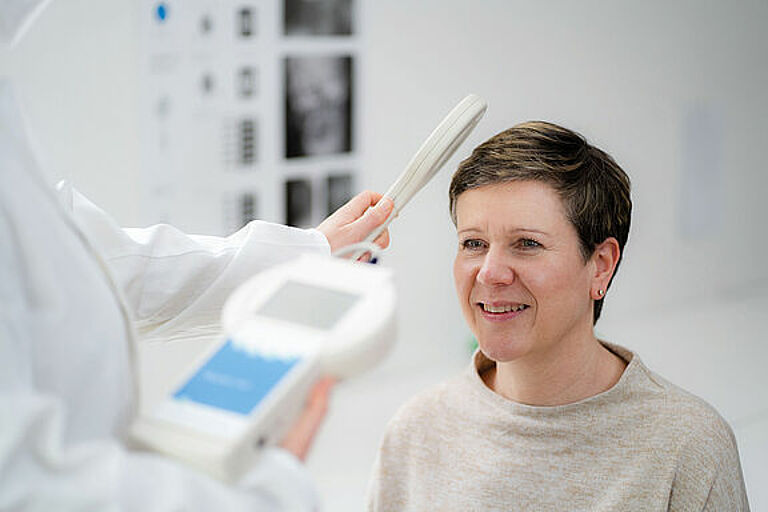
With the antenna of the associated external reader unit set held over the implanted M.scio, the pressure within the shunt (i.e., usually between the tip of the ventricular catheter and the first differential pressure valve) can be determined. The internal shunt pressure (ISP) thus measured is not directly equivalent to the intraventricular pressure (IVP). However, the latter is transmitted directly from the ventricles to the M.scio through a short piece of fluid-filled silicone catheter and is therefore closely related to it. Apart from minimal attenuation/delay of rapid, dynamic IVP progressions, this arrangement causes the pressure measured by the M.scio to be several cmH2O lower than the true IVP when the patient is standing, because a hydrostatic fluid column forms between the M.scio and the ventricles. However, in the supine position the ventricle (or the reference point, the foramina of Monro) and the M.scio are at comparable heights, and the measured pressure is then relatively equal to the IVP. For the sake of completeness, it should be mentioned that when the valve is open, CSF (cerebrospinal fluid) moves past the measuring cell through the shunt; in this case a minimal dynamic pressure correction via Bernoulli’s equation would still have to be taken into account. However, this theoretically possible and simple derivation of the IVP from the value measured by the M.scio does not play a significant role in shunt function control (SFK).
The following examples 1–7 are therefore only intended to illustrate theoretically possible procedures and no responsibility can be assumed for their practical implementation. The responsibility lies exclusively with the attending physician.
1. In case of intentionally induced hyperventilation of the patient, a rapid pressure drop in the ventricles and in the shunt should be measurable. Otherwise, occlusion or disconnection of the proximal connection between M.scio and ventricles is likely.
2. A similar idea is behind performing the Valsalva maneuver, in which patients breathe/press against the occluded nasal and oral orifices. If the M.scio and ventricle are properly connected, a rapid increase in pressure should be measurable.
3. Such an immediate rise of pressure should also result from lowering the head or ventricles from the recumbent position below the level of the spinal column, e.g., by tilting the bed accordingly (in the direction of a “headstand”). This, too, shows the undisrupted function of the ventricular catheter.
4. If in doubt, the physician may decide to briefly lower the valve setting while the patient is standing to produce the desired effect of “artificial overdrainage”. If this effect is visible, the shunt can be assumed to be distally unobstructed. The reason for this is that the overdrainage suction can only form if the fluid column in the distal catheter part is able to move freely and not inhibited by any occlusion.
In general, it can be assumed that an adjustment of the valve opening pressure will not show up in the pressure measurement in such a simple, linear way as suggested by the simplified hydrostatic bucket model.30,31
5. Nevertheless, any relevant adjustment of a functioning shunt (i.e., an adjustment of at least a few cmH2O) should also be reflected in changes in the measured values in the short term; otherwise, there is a probability of a shunt malfunction. How exactly the adjustment of a properly functioning shunt affects the actual ICP is the subject of more recent research.32,33
The aforementioned necessary conditions for SFC are effortlessly fulfilled by the M.scio. After all, with up to 44 single measurements per second, it achieves a sampling rate previously unattainable for telemetric in-situ pressure sensors. Unfortunately, this unique feature has been erroneously and misleadingly described in recent literature as a maximum temporal resolution of 1 Hz instead of 44 Hz.34 With 44 Hz, the M.scio can not only visualize the P wave (pulse) induced by the heartbeat, but even display its fine structure.
Figure 1 shows a typical fast measurement with the M.scio, in which the comparatively high-frequency P wave of cardiac origin (pulse wave, purple) and the slower respiratory-induced R wave (respiratory wave, green) are clearly visible. Figure 2 shows that the P wave itself still has a fine structure consisting of (at least) three different maxima, which is perfectly discernible in a fast measurement with the M.scio. The P wave is generated by the pulsatile pressure fluctuation of the arterial blood supply to the brain, which is transmitted to the cerebrospinal fluid by various pathways, mainly via the choroid plexus, the subarachnoid space, and the arterial capillary bed.
Figure 1: Example of an M.scio fast measurement with 44 Hz sampling rate, clearly showing an overlay of the pulse-induced P waves (purple) and the slower respiration-induced R waves (green).
Figure 2: Magnified section of the pressure curve recorded with the M.scio. With a maximum temporal resolution of 44 Hz, M.scio measurement data can be used to determine the P wave amplitude and to visualize its fine structure (namely the peaks P1, P2, and P3).
6. Therefore, the P wave should be visible in any fast measurement with M.scio; otherwise, an occluded or severed proximal connection to the ventricles is likely.
Although the amplitude of the P wave varies markedly, depending, among other things, on intracranial compliance (link), and thus conveys important diagnostic information (link), complete disappearance (i.e., amplitude = 0) is usually not possible. 35-37 Therefore, such a fast measurement with the M.scio makes it particularly simple to perform a robust functional check of the proximal part of the shunt, even without any medical manipulation or assistance of the patient. In other words, visible P waves indicate patency of the shunt between the ventricle and the M.scio.
7. Theoretically, it is possible that the implantation procedure reduces the intracranial CSF volume due to CSF loss and that intracranial compliance is thus temporarily increased (i.e., shortly after surgery). This would make the pulsations in the pressure signal of the M.scio impossible or difficult to detect. The test from example 2 above could provide a remedy here, since a Valsalva maneuver would generally cause larger pressure changes than P waves, thus making it possible to detect a change in morphology.
From these observations and considerations, it becomes obvious that
- on the one hand, shunt function controls are an important but not yet standardized area to be observed and further developed.
- on the other hand, the M.scio has potential for various applications. Conceivable and described in initial studies are, for example
- Measurement of intracranial pressure17
- pressure measurement in stand-alone mode, i.e., not as part of a shunt17
- shunt adjustment based on pressure measurements9, 18
- obtaining parameters such as the amplitude of P waves and their fine structure, from which therapeutic/diagnostic information about intracranial compliance and other aspects could be gained39
To reflect the real-world, full-scale use of M.scio in clinical practice, we conducted a user survey, which is presented below.
The neurosurgeons contacted for the survey were mostly selected by how many M.scios they had implanted so far (highest numbers). The intent was to capture the largest possible number of implanted products along with the most extensive user experience. If they agreed to participate, these “power users” were presented with a 4-page questionnaire containing the following 12 questions (see below for summary).
Note
At the time the survey was conducted, the product name of the M.scio was still SENSOR RESERVOIR or SENSOR PRECHAMBER. Since the beginning of 2020, their names are now M.scio, dome-angled and dome-inline, and the two variants M.scio, flat-angled and flat-inline, respectively, have been added to the product line.
The questionnaire was then completed, signed, and returned to us for evaluation.
1. How many of each product variant – SENSOR RESERVOIR or SENSOR PRECHAMBER – have you implanted to date?
2. In which patient groups have you mainly implanted the SENSOR RESERVOIR/SENSOR PRECHAMBER ?
3. For which specific reasons have you implanted the SENSOR RESERVOIR/SENSOR PRECHAMBER?
4. Do you prefer one of the two product variants (RESERVOIR or PRECHAMBER) and why?
5. Were you able to perform a shunt function check (SFC) by means of measurement with the SENSOR RESERVOIR or the SENSOR PRECHAMBER?
6. Related to question 5: Were the measurements helpful for other purposes as well?
7. How many implants have been functioning without complications since their implantation?
Questions 8–10: To be answered only if a malfunction or complication related to the SENSOR RESERVOIR/SENSOR PRECHAMBER has occurred.
8. In how many SENSOR RESERVOIRS/SENSOR PRECHAMBERS did malfunctions orn complications occur? Which complications or malfunctions?
9. After what timeframe did the complications occur on average?
10. Were any of the mentioned complications or malfunctions the reason for a revision of the SENSOR RESERVOIR/SENSOR PRECHAMBER or the entire shunt system?
11. Do you have any suggestions or requests for improvement of the SENSOR RESERVOIR/SENSOR PRECHAMBER?
12. What is your general evaluation or final comment on the SENSOR RESERVOIR/SENSOR PRECHAMBER?
All 19 questionnaires we sent out were returned completed, from 18 different clinics in the UK, Germany, Italy, Spain, and the Czech Republic. The data we obtained were from implantations in all patient groups without exception, from preterm infants through children and adults to elderly NPH patients.
All questionnaires were consistent, so that no data had to be excluded from the analysis. The total sample covered a size of 284 implants, which represents a high proportion of the device population. The survey of “power users” generally provides more data, and from experienced users who are better able to assess the advantages and disadvantages, the performance, and the possible hazards of using the M.scio than those with only sporadic experience. Therefore, we are confident that these data provide a valid and reliable overall picture of M.scio and its clinical use (see Figure 3).
Figure 3 shows the main results of the survey at a glance:
Figure 3: Overview of the main results of our M.scio user survey.
The survey includes 284 implants. When asked about their general assessment (question 12), 100% of all surveyed users rated the M.scio as helpful, more specifically: 44% as rather helpful and 56% as very helpful (middle). Moreover, 95% of the surveyed users were able to successfully perform a shunt function control in the vast majority of cases – question 5. Results on longevity (question 7) are reflected in Figure 3 (right): 26% of the 284 implants had been implanted for less than one year at the time of the survey, 19% between one and two years, 13% between two and three years, 38% even longer than three years. 4% of the implants were revised because of shunt problems that were not demonstrably caused by the M.scio (i.e., infection, occlusion). All of these M.scio units (99% of the total sample) functioned flawlessly after implantation or even after revision and showed no signs of failure.
The answers to questions 8–10, which cover all electronic, mechanical, and other malfunctions, also correspond with this finding. Except for three temporary/reversible error messages (e.g., temperature error message on the reader unit set display), no other problems were reported, especially none that were a reason for revisions.
1% of M.scios (three units) were revised because of infections. They had been caused by implant-induced wound healing problems, with two of them affecting the same patient. In fact, the traditional M.scio (variants “dome-angled” and “dome-inline”) examined during the survey has a comparatively large height of 7.75 mm; it has already been discussed in literature that this can be problematic for sensitive patients or infants.9 Antes et al. describe a rate of 2 in 25, i.e., 8% for such wound healing disorders.9 A complication rate of 1–8% may not be an unacceptably high risk given the high benefit, but 79% of the surveyed physicians clearly expressed their demand for a lower implant height as part of questions 11 & 12 (cf. Rot, Dweek, Gutowski, Goelz, Meier and Lemcke35 2020). We have responded to this request and developed two variants with a 40% reduced height of now 4.8 mm. They have been approved and are available under the variant designations “flat-angled” and “flat-inline” since the beginning of 2020.
In the entire time since approval, only three total failures of the measuring cell (i.e., a complete irreversible loss of the electronic measuring function) had been reported. However, as already mentioned, the M.scio does not have to be revised because of this issue; instead, it remains in patients, e.g., to be used as a normal reservoir or as a connector.

Another important requirement emerged in the context of the user wishes expressed in questions 11 & 12. It is outlined by the following keywords from the survey: “long duration measurement”, “continuous monitoring”, “home monitoring/measurement”, “head fixation or helmet”, “smaller antenna”, “smaller reader”. This complex set of demands was requested or indicated by 47% of the users. This seems incomprehensible at first, since the M.scio already offers a long-term measurement mode, which theoretically makes measurements possible over several months (only limited by the capacity of the memory card). According to our analyses, this is really mainly about the (sometimes explicitly expressed) need for a more handy antenna and a smaller reader to facilitate a permanent head fixation. This should make long-term pressure measurements possible in a stationary environment as well as in everyday surroundings (e.g., in home monitoring). This demand is also reflected in the literature.39 We do not agree with the statement of Norager et al. that a fixation of the antenna at the head is impossible, because this was demonstrably carried out in clinical practice. However, it is indisputable that such a permanent fixation is difficult and uncomfortable so far.
This demand by almost 50% of the users clearly shows their expectation and partly also their practice that the M.scio is not only used for relative and short-term pressure measurements in the context of SFC, but also as a “brain pressure probe” for long-term measurements of absolute values.
Questions 3 and 6 specifically address this issue of expanded application. They ask about the original reasons for implanting the M.scio and about its actual usefulness in additional applications other than shunt function controls. It is clear that 100% of users also utilize the M.scio for additional off-label applications. The significant keywords mentioned in the survey are: “complex/demanding patient/hydrocephalus”, “valve/shunt adjustment”, “ICP measurement”, but also “therapy support” and “research”. Except for the last two keywords, all others have in common that, according to our analyses, they are ultimately always about intracranial pressure measurement. The group of patients often colloquially referred to as “complex/demanding patients” initially includes those whose symptoms are diffuse or nonspecific and cannot be relieved by simple valve adjustment. As recently described by Antes et al. and Pennacchietti et al., in such difficult cases the estimation of ICP from M.scio measurements may prove helpful in determining the direction and extent of the required valve adjustment.9,17 As mentioned above, the naive linear hydrostatic bucket model is not very useful for an estimation of this type. 30,31 Norager et al. also describe a use of the M.scio for ICP measurement as a guideline for valve adjustment.39 In this case review, a control calibration by puncturing the reservoir was also performed at the same time, successfully demonstrating the required absolute accuracy and absence of drift of the M.scio. The group of “complex/demanding patients” also includes IIH patients, whose special treatment was described for example by Thompson.40 In addition to the possibility of pressure measurement-assisted valve adjustment, Thompson points out in this publication that “telemetric measuring of patients with IIH has the potential to become routine in the years to come” due to its multiple benefits, including the “positive subjective feedback from patients and their families.”40 The point here is that this group of patients is known to develop symptoms that are not directly related to an intracranial pressure crisis. A simple examination by the physician showing that the intracranial pressure is within the normal range, or at least unchanged since the last examination, often has a significant positive therapeutic effect here.
In a recently published retrospective study of 81 patients, Galloway et al. showed that implementation of a standard protocol in shunting IIH patients significantly reduced the revision rate from 22% to 6% within the first 30 days after surgery.41 Among other things, the protocol recommends implantation of an adjustable gravitational valve as well as the M.scio for ICP monitoring. This can be considered the first positive confirmation of the great potential of telemetric intracranial pressure measurement in IIH patients as predicted by Thompson.
The use of the M.scio in the so-called "stand-alone mode" reported by users also aims to determine intracranial pressure. In this case, the M.scio is not part of a (CSF-perfused) shunt, instead it is implanted independently. The proximal end is connected to the ventricles (or, for example, a brain cyst) as usual, but the distal end has a blank closure. The pressure to be measured is transmitted to the M.scio through the fluid-filled ventricular catheter just as in the shunt-integrated application. Therefore, the calculation of the ICP must also consider the position and size of the additional hydrostatic column in the ventricular catheter, but a dynamic correction (as per Bernoulli) is not necessary. This type of application was described three times in our survey, i.e. by 16% of the users, and we are also aware of this use from other sources.17,39,42,43 If it becomes necessary, e.g., if a pathological overpressure is detected/measured, this configuration can easily be extended to a shunt by additionally implanting a valve with a distal peritoneal catheter.
Approval extension
Based on the very positive results of the PMCF survey and the wide use of the M.scio described in the literature, it was possible to expand the intended use of the product to include diagnostic intracranial pressure measurement within the cerebrospinal fluid.
The 2019 user survey presented here showed that 95% of all surveyed users were able to realize the shunt function control with the M.scio in most cases, with 56% finding the implant very useful and 44% finding it rather useful. Apart from the comparatively tall height of the variant dome, which can lead to wound healing disorders in sensitive and young patients, no other risks were identified. To address this risk, two variants that are 40% flatter were approved in early 2020. 38% of implants have been proven to function without failure for more than three years. 13% had been implanted without issue for two to three years at the time of the survey, 19% between one and two years, and 26% for less than one year. There is no indication that malfunctions are to be expected with them in the future. In general, only three complete failures have been documented since approval in 2015, and there are no known relevant function-limiting factors such as aging or wear.
In particular, proper function, especially the patency of the proximal connection from ventricular catheter to the M.scio, can be easily and safely verified by the visibility of the P wave. Nevertheless, overall shunt function checks with the M.scio do not yet follow a standardized protocol, and further clinical experience is needed to develop such a procedure. Scientific publications on this topic are needed and desired.
The survey shows, as does the literature, that in practice, the M.scio is being widely used beyond its existing intended purpose. The most important extended use is ICP measurement and valve setting optimization on that base. ICP measurement is even performed in stand-alone mode. The reference pressure measurements for accuracy and absence of drift, performed by users on their own responsibility, suggest that such an application is justified, safe, and useful.
Overall, the risk-benefit ratio is positive and more than justifiable. In addition to its application for shunt function controls, the M.scio shows a clear potential as a research tool. It could be used in the future to determine or investigate further diagnostic and therapeutic parameters such as intracranial compliance based on the pressure curves and their fine structure.

1. Dunn LT. Raised intracranial pressure. 2002, J Neurol Neurosurg Psychiatry. 2002;73(1):i23-7. DOI:10.1136/jnnp.73.suppl_1.
2. Huttner H. Intrakranieller Druck (ICP), S1-Leitlinie, 2018. Deutsche Gesellschaft für Neurologie (Hrsg.), Leitlinien für Diagnostik und Therapie in der Neurologie. [cited 2022 Jan 12]. Available from: URL: www.dgn.org/leitlinien.
3. Le Roux P. Intracranial Pressure Monitoring and Intracranial Pressure Monitoring and Management. In: Laskowitz D, Grant G, editors. Translational Research in Traumatic Brain Injury; Boca Raton (FL): CRC Press/Taylor and Francis Group, 2016. Chapter 15.
4. Evensen KB, Eide PK. Measuring intracranial pressure by invasive, less invasive or non-invasive means: limitations and avenues for improvement. Fluids Barriers CNS. 2020 May 6;17(1):34. DOI:10.1186/s12987-020-00195-3.
5. Yu L, Kim BJ, Meng E. Chronically implanted pressure sensors: challenges and state of the field. Sensors (Basel). 2014 Oct 31;14(11):20620–20644. DOI:10.3390/s141120620.
6. Nag DS, Sahu S, Swain A, Kant S. Intracranial pressure monitoring: Gold standard and recent innovations. World J Clin Cases. 2019 Jul 6;7(13):1535-1553.
7. Kawoos U, McCarron R M, Auker CR, Chavko M. Advances in Intracranial Pressure Monitoring and Its Significance in Managing Traumatic Brain Injury. Int J Mol Sci. 2015 Dec 4;16(12):28979-28997.
8. Frischholz M, Sarmento L, Wenzel M, Aquilina K, Edwards R, Coakham HB. Telemetric implantable pressure sensor for short- and long-term monitoring of intracranial pressure. Conf Proc IEEE Eng Med Biol Soc. 2007:514.
9. Antes S, Stadie A, Muller S, Linsler S, Breuskin D, Oertel J. Intracranial Pressure-Guided Shunt Valve Adjustments with the Miethke Sensor Reservoir. World Neurosurg. 2018 Jan;109:e642-e650. DOI: 10.1016/j.wneu.2017.10.044 .
10. London Health Science Center. PROCEDURE: INSERTION OF CODMAN MICROSENSOR EVD OR INTRAPARENCHYMAL MONITOR AND SETUP OF CODMAN EXPRESS [Internet]. London: London Health Science Center; 2008 Jun 12 [Reviewed 2022 Jan 20]. Available from:
11. Pedersen SH, Lilja-Cyron A, Astrand R, Juhler M. Monitoring and Measurement of Intracranial Pressure in Pediatric Head Trauma. Front Neurol. 2020 Jan 14;10:1376. doi: 10.3389/fneur.2019.01376.
12. Eide PK, Bakken A. The baseline pressure of intracranial pressure (ICP) sensors can be altered by electrostatic discharges. Biomed Eng Online. 2011 Aug 22;10:75. doi: 10.1186/1475-925X-10-75.
13. Czosnyka M, Czosnyka Z. Origin of intracranial pressure pulse waveform. Acta Neurochir (Wien). 2020 Aug;162(8):1815-1817. doi: 10.1007/s00701-020-04424-4.
14. Turtz AR. Fiberoptic lntracranial Pressure Monitors. Intracranial Pressure Monitoring Device In: Daroff R, Aminoff M, editors. Encyclopaedia of the Neurological Sciences (Second Edition). Amsterdam et al: Academic Press; 2014.
15. Anderson RC, Kan P, Klimo P, Brockmeyer DL, Walker ML, Kestle JR. Complications of intracranial pressure monitoring in children with head trauma. J Neurosurg. 2004 Aug;101(1 Suppl):53-8. doi: 10.3171/ped.2004.101.2.0053.
16. Raboel PH, Bartek J Jr, Andresen M, Bellander BM, Romner B. Intracranial Pressure Monitoring: Invasive versus Non-Invasive Methods-A Review. Crit Care Res Pract. 2012;2012:950393. doi: 10.1155/2012/950393.
17. Pennacchietti V, Prinz V, Schaumann A, Finger T, Schulz M, Thomale UW. Single center experiences with telemetric intracranial pressure measurements in patients with CSF circulation disturbances. Acta Neurochir (Wien). 2020 Oct;162(10):2487-2497. doi: 10.1007/s00701-020-04421-7.
18. Bjornson A, Henderson D, Lawrence E, McMullan J, Ushewokunze S. The Sensor Reservoir-does it change management? Acta Neurochir (Wien). 2021 Apr;163(4):1087-1095. doi: 10.1007/s00701-021-04729-y.
19. Merkler AE, Ch'ang J, Parker WE, Murthy SB, Kamel H. The Rate of Complications after Ventriculoperitoneal Shunt Surgery. World Neurosurg. 2017 Feb;98:654-658. doi: 10.1016/j.wneu.2016.10.136.
20. Bock HC, Kanzler M, Thomale UW, Ludwig HC. Implementing a digital real-time Hydrocephalus and Shunt Registry to evaluate contemporary pattern of care and surgical outcome in pediatric hydrocephalus. Childs Nerv Syst. 2018 Mar;34(3):457-464. doi: 10.1007/s00381-017-3654-0.
21. Beuriat PA, Puget S, Cinalli G, Blauwblomme T, Beccaria K, Zerah M, Sainte-Rose C. Hydrocephalus treatment in children: long-term outcome in 975 consecutive patients. J Neurosurg Pediatr. 2017 Jul;20(1):10-18. doi: 10.3171/2017.2.PEDS16491.
22. Lalou AD, Czosnyka M, Garnett MR, Nabbanja E, Petrella G, Hutchinson PJ, Pickard JD, Czosnyka Z. Shunt infusion studies: impact on patient outcome, including health economics. Acta Neurochir (Wien). 2020 May;162(5):1019-1031. doi: 10.1007/s00701-020-04212-0.
23. Aralar A, Bird M, Graham R, Koo B, Chitnis P, Sikdar S, Shenai M. Assessment of Ventriculoperitoneal Shunt Function Using Ultrasound Characterization of Valve Interface Oscillation as a Proxy. Cureus. 2018 Feb 19;10(2):e2205. doi: 10.7759/cureus.2205.
24. Rocque BG, Lapsiwala S, Iskandar BJ. Ventricular shunt tap as a predictor of proximal shunt malfunction in children: a prospective study. J Neurosurg Pediatr. 2008 Jun;1(6):439-43. doi: 10.3171/PED/2008/1/6/439.
25. Schlosser HG, Crawack HJ, Miethke C, Knitter T, Zeiner A, Sprung C. An improved reservoir for the flushing test to diagnose shunt insufficiency. Neurosurg Focus. 2016 Sep;41(3):E14. doi: 10.3171/2016.6.FOCUS15540.
26. Boyle TP, Nigrovic LE. Radiographic evaluation of pediatric cerebrospinal fluid shunt malfunction in the emergency setting. Pediatr Emerg Care. 2015 Jun;31(6):435-40; quiz 441-3. doi: 10.1097/PEC.0000000000000462.
27. Boyle TP, Paldino MJ, Kimia AA, Fitz BM, Madsen JR, Monuteaux MC, Nigrovic LE. Comparison of rapid cranial MRI to CT for ventricular shunt malfunction. Pediatrics. 2014 Jul;134(1):e47-54. doi: 10.1542/peds.2013-3739.
28. Meulepas JM, Ronckers CM, Smets AMJB, Nievelstein RAJ, Gradowska P, Lee C, et al. Radiation Exposure From Pediatric CT Scans and Subsequent Cancer Risk in the Netherlands. J Natl Cancer Inst. 2019 Mar 1;111(3):256-263. doi: 10.1093/jnci/djy104.
29. Koueik J, Kraemer MR, Hsu D, Rizk E, Zea R, Haldeman C, Iskandar BJ. A 12-year single-center retrospective analysis of antisiphon devices to prevent proximal ventricular shunt obstruction for hydrocephalus. J Neurosurg Pediatr. 2019 Sep 6:1-10. doi: 10.3171/2019.6.PEDS1951.
30. Kombogiorgas D. Cerebrospinal Fluid Shunts. New York: Nova Science; 2016.
31. Fritsch M, Kehler U and Meier U. Normal Pressure Hydrocephalus: Pathophysiology Diagnosis Treatment. Stuttgart et al: Thieme; 2014.
32. Farahmand D, Qvarlander S, Malm J, Wikkelsö C, Eklund A, Tisell M. Intracranial pressure in hydrocephalus: impact of shunt adjustments and body positions. J Neurol Neurosurg Psychiatry. 2015 Feb;86(2):222-8. doi: 10.1136/jnnp-2014-307873.
33. D'Antona L, Craven CL, Merchan MAJ, Thompson SD, Bremner F, Thorne L, Matharu MS, Watkins LD, Toma AK. Paradoxical response of intracranial pressure to shunt valve setting adjustments. Acta Neurochir (Wien). 2020 Oct;162(10):2451-2458. doi: 10.1007/s00701-020-04462-y.
34. Ertl P, Hermann EJ, Heissler HE, Krauss JK. Telemetric Intracranial Pressure Recording via a Shunt System Integrated Sensor: A Safety and Feasibility Study. J Neurol Surg A Cent Eur Neurosurg. 2017 Nov;78(6):572-575. doi: 10.1055/s-0037-1603632.
35. Holm S, Eide PK. Impact of sampling rate for time domain analysis of continuous intracranial pressure (ICP) signals. Med Eng Phys. 2009 Jun;31(5):601-6. doi: 10.1016/j.medengphy.2008.12.001.
36. Czosnyka M, Czosnyka Z, Keong N, Lavinio A, Smielewski P, Momjian S, Schmidt EA, Petrella G, Owler B, Pickard JD. Pulse pressure waveform in hydrocephalus: what it is and what it isn't. Neurosurg Focus. 2007 Apr 15;22(4):E2. doi: 10.3171/foc.2007.22.4.3.
37. Schuhmann MU, Sood S, McAllister JP, Jaeger M, Ham SD, Czosnyka Z, Czosnyka M. Value of overnight monitoring of intracranial pressure in hydrocephalic children. Pediatr Neurosurg. 2008;44(4):269-79. doi: 10.1159/000131675.
38. Rot S, Dweek M, Gutowski P, Goelz L, Meier U, Lemcke J. Comparative investigation of different telemetric methods for measuring intracranial pressure: a prospective pilot study. Fluids Barriers CNS. 2020 Oct 17;17(1):63. doi: 10.1186/s12987-020-00225-0.
39. Norager NH, Lilja-Cyron A, Hansen TS, Juhler M. Deciding on Appropriate Telemetric Intracranial Pressure Monitoring System. World Neurosurg. 2019 Jun;126:564-569. doi: 10.1016/j.wneu.2019.03.077.
40. Thompson S. D.: Management of idiopathic intracranial hypertension: treatment options and advances. 2017, British Journal of Neuroscience Nursing. 2017 Sep 1;13(4):187-191. doi: doi.org/10.12968/bjnn.2017.13.4.187.
41. Galloway L, Karia K, White AM, Byrne ME, Sinclair AJ, Mollan SP, Tsermoulas G. Cerebrospinal fluid shunting protocol for idiopathic intracranial hypertension for an improved revision rate. J Neurosurg. 2021 Oct 8:1-6. doi: 10.3171/2021.5.JNS21821.
42. D’Antona L, Craven CL, Thompson S, Sennik S, Asif H, Mediratta S, et al. Telemetric ICP monitoring after neuroendoscopic treatment of hydrocephalus: a valuable tool to recognize treatment failure In: Abstracts from Hydrocephalus 2018: the tenth meeting of the International Society for Hydrocephalus and Cerebrospinal Fluid Disorders. Fluids Barriers CNS. 2018 Dec 24;15(Suppl 2). Doi: doi.org/10.1186/s12987-018-0119-0
43. Craven CL, Mullins SR, Asif H, D’Antona L, Thompson S, Watkins LD, Toma AK, Horsley V. CSF dynamics following endoscopic repair of anterior skull base defects In: Abstracts from Hydrocephalus 2019: The Eleventh Meeting of the International Society for Hydrocephalus and Cerebrospinal Fluid Disorders. Fluids Barriers CNS. 2019 Dec 5;16(Suppl 3). Doi: doi.org/10.1186/s12987-019-0156-3